Synchrotron radiation
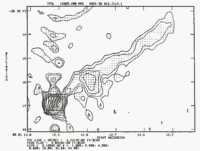
Synchrotron radiation is radiation produced from particles traveling along curved paths near the speed of light.
Radiation
"Synchrotron radiation is the electromagnetic radiation emitted when charged particles travel in curved paths. Because in most accelerators the particle trajectories are bent by magnetic fields, synchrotron radiation is also called Magneto-Bremsstrahlung. The emitted spectrum is broadband from the microwave (harmonics of the driving RF field) to x-ray spectral regions. The radiation is vertically collimated and polarized. The synchrotron radiation output can be calculated if the electron energy, bending radius, electron current, angle relative to the orbital plane, the distance to the tangent point and vertical and horizontal acceptance angles are known."[1]
Cyclotron radiation
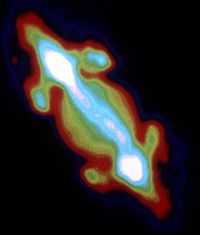
Forms of decametric radio signals from Jupiter:
- "bursts (with a wavelength of tens of meters) vary with the rotation of Jupiter, and are influenced by interaction of Io with Jupiter's magnetic field.[2]"[3]
- "emission (with wavelengths measured in centimeters) was first observed by Frank Drake and Hein Hvatum in 1959.[4] The origin of this signal was from a torus-shaped belt around Jupiter's equator. This signal is caused by cyclotron radiation from electrons that are accelerated in Jupiter's magnetic field.[5]"[3]
Theoretical synchrotron radiation
Def. "the electromagnetic radiation emitted by the accelerating charged particles in a synchrotron that are moving at near the speed of light"[6] is called synchrotron radiation.
"We now assume that the γ-rays are produced [from 3C 279] by relativistic electrons via Compton scattering of synchrotron photons (SSC). In any such model, the fact that the γ-rays luminosity, produced via Compton scattering, is higher than that emitted at lower frequencies (1014 - 1016 Hz), supposedly via the synchrotron process, implies a radiation energy density, Ur, higher than the magnetic energy density, UB. From the observed power ratio we derive that Ur must be one order of magnitude greater than UB, which may be a lower limit if Klein-Nishina effects reduce the efficiency of the self-Compton emission. This result is independent of the degree of beaming, which, for a homogeneous source, affects both the synchrotron and the self-Compton fluxes in the same way. This source is therefore the first observed case of the result of a Compton catastrophe (Hoyle, Burbidge, & Sargent 1966)."[7]
Objects
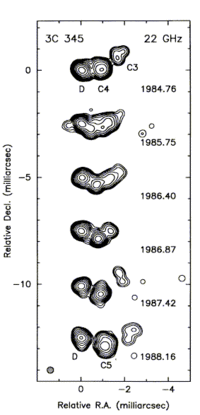
"Tracking the features within small-scale jets has revealed interesting complications. The paths are not always radial to the nucleus, usually taken as the source with the flattest spectrum in ambiguous cases. This is based on the general principle that synchrotron spectra are flattened at lower frequencies by self-absorption, so the densest plasma will have a flat or inverted spectrum. An interesting case is 3C 345 [at right], in which emission features repeatedly appear off the core and follow fairly consistent nonradial paths. [...] a new component appears in late 1985, brightens, and moves outward changing its relative position angle in the process".[8]
Electromagnetics
"The magnetic field is well-ordered in many jets, as shown by polarization measurements. Synchrotron radiation can be very highly polarized (50%) if the field is globally ordered, and some sources [approach] this level. The electric vectors show clear structure and alignment; an especially common pattern is for the field lines to be along the jet in the inner portions and transition to an azimuthal configuration farther out. This is seen in [PKS0521-36 at 2 cm]."[8]
Continuum
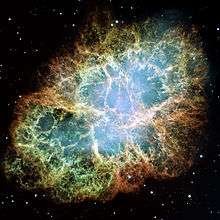
Like X-rays, the gamma-ray "continuum can arise from bremsstrahlung, black-body radiation, synchrotron radiation, or what is called inverse Compton scattering of lower-energy photons by relativistic electrons, knock-on collisions of fast protons with atomic electrons, and atomic recombination, with or without additional electron transitions.[9]"[10]
The radio continuum from an active galactic nucleus "is always due to a jet. It shows a spectrum characteristic of synchrotron radiation."[11]
"[T]he diffuse blue region is predominantly produced by synchrotron radiation, which is radiation given off by the curving motion of electrons in a magnetic field. The radiation corresponded to electrons moving at speeds up to half the speed of light."[12]
A synchrotron model for the continuum spectrum of the Crab Nebula fits the radiation given off.[13]
"In the Crab Nebula X-ray spectrum there are three features that differ greatly from Scorpius X-1: its spectrum is much harder, its source diameter is in light-years (ly)s, not astronomical units (AU), and its radio and optical synchrotron emission are strong.[9] Its overall X-ray luminosity rivals the optical emission and could be that of a nonthermal plasma. However, the Crab Nebula appears as an X-ray source that is a central freely expanding ball of dilute plasma, where the energy content is 100 times the total energy content of the large visible and radio portion, obtained from the unknown source.[9]"[10]
Protons
"Radio observations at 210 GHz taken by the Bernese Multibeam Radiometer for KOSMA (BEMRAK) [...] at submillimeter wavelengths [show an impulsive component that] starts simultaneously with high-energy (>200 MeV nucleon−1) proton acceleration and the production of pions. The derived radio source size is compact (≤10"), and the emission is cospatial with the location of precipitating flare-accelerated >30 MeV protons as seen in γ-ray imaging."[14]
"In the [Large Hadron Collider] LHC proton bunches also produce [synchrotron] radiation at increasing amplitude and frequency as they accelerate with respect to the vacuum field, propagating photoelectrons, which in turn propagate secondary electrons from the pipe walls with increasing frequency and density up to 7x1010. Each proton may lose 6.7keV per turn due to this phenomenon.[15]"[16]
Electrons
"Radio observations at 210 GHz taken by the Bernese Multibeam Radiometer for KOSMA (BEMRAK) [of] high-energy particle acceleration during the energetic solar flare of 2003 October 28 [...] at submillimeter wavelengths [reveal] a gradual, long-lasting (>30 minutes) component with large apparent source sizes (~60"). Its spectrum below ~200 GHz is consistent with synchrotron emission from flare-accelerated electrons producing hard X-ray and γ-ray bremsstrahlung assuming a magnetic field strength of ≥200 G in the radio source and a confinement time of the radio-emitting electrons in the source of less than 30 s. [... There is a] close correlation in time and space of radio emission with the production of pions".[14]
Gamma rays
A "fading counterpart to GRB 980329 at 850 μm [has been found]. [...] the sub-millimeter flux was relatively bright. [...] The radio through sub-millimeter spectrum of GRB 980329 is well fit by a power law with index α = +0.9. However, we cannot exclude a ν1/3 power law attenuated by synchrotron self-absorption."[17]
X-rays
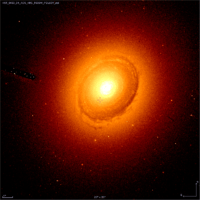
"NGC 383 is a double radio galaxy[3] with a quasar-like appearance located in the constellation Pisces. [...] Recent discoveries by the National Radio Astronomy Observatory in 2006 reveal that NGC 383 is being bisected by high energy relativistic electrons traveling at relatively high fractions of the speed of light. These relativistic electrons are detected as synchrotron radiation in the x-ray and radio wavelengths. The focus of this intense energy is the galactic center of NGC 383. The relativistic electron jets detected as synchrotron radiation extend for several thousand parsecs and then appear to dissipate at the ends in the form of streamers or filaments."[18]
Blues
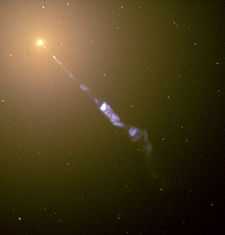
The image at right shows the blue light, towards the lower right, due to synchrotron radiation, of a jet emerging from the bright active galactic nucleus (AGN) core of Messier 87.
Submillimeters
Submillimeter observations
- determine "the breaks in the radio to sub-millimeter to optical spectrum so that the spectral shape can be compared to the synchrotron models"[17]
- determine "the evolution of the sub-millimeter flux"[17] and
- look "for underlying quiescent sources that may be dusty star-forming galaxies at high redshifts."[17]
Radios
“Although some radio waves are produced by astronomical objects in the form of thermal emission, most of the radio emission that is observed from Earth is seen in the form of synchrotron radiation, which is produced when electrons oscillate around magnetic fields.[19]"[20]
Earth
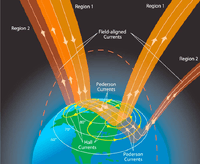
"Electrons moving along a Birkeland current may be accelerated by a plasma double layer. If the resulting electrons approach relativistic velocities (i.e. if they approach the speed of light) they may subsequently produce a Bennett pinch, which in a magnetic field causes the electrons to spiral and emit synchrotron radiation that may include radio, optical (i.e. visible light), x-rays, and gamma rays."[21]
Jupiter
"This VLA image of Jupiter [at right] doesn't look like a planetary disk at all. Most of the radio emission is synchrotron radiation from electrons in Jupiter's magnetic field."[22]
Galaxies
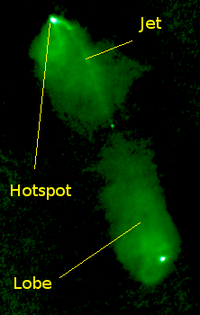
"The hotspots that are usually seen in FRII sources are interpreted as being the visible manifestations of shocks formed when the fast, and therefore supersonic, jet (the speed of sound cannot exceed c/√3) abruptly terminates at the end of the source, and their spectral energy distributions are consistent with this picture.[23]"[24]
Mathematics
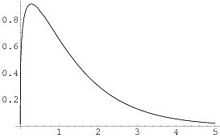
Ions accelerating past each other at relativistic speeds generate synchrotron radiation. Depending on the ion concentration, this radiation may contribute significantly to stellar spectra as may cyclotron or bremsstrahlung radiation from similar ion and electron interactions at slower speeds.
"In astrophysics, x is usually a ratio of frequencies, that is, the frequency over a critical frequency (critical frequency is the frequency at which most synchrotron radiation is radiated). This is needed when calculating the spectra for different types of synchrotron emission. It takes a spectrum of electrons (or any charged particle) generated by a separate process (such as a power law distribution of electrons and positrons from a constant injection spectrum) and converts this to the spectrum of photons generated by the input electrons/positrons.”[25]
Technology
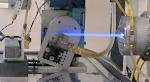
“A synchrotron is a particular type of cyclic particle accelerator originating from the cyclotron in which the guiding magnetic field (bending the particles into a closed path) is time-dependent, being synchronized to a particle beam of increasing kinetic energy. The synchrotron is one of the first accelerator concepts that enable the construction of large-scale facilities, since bending, beam focusing and acceleration can be separated into different components. ... Unlike in a cyclotron, synchrotrons are unable to accelerate particles from zero kinetic energy; one of the obvious reasons for this is that its closed particle path would be cut by a device that emits particles. Thus, schemes were developed to inject pre-accelerated particle beams into a synchrotron. The pre-acceleration can be realized by a chain of other accelerator structures like a linac, a microtron or another synchrotron; all of these in turn need to be fed by a particle source comprising a simple high voltage power supply, typically a Cockcroft-Walton generator.”[25]
At the right is an image that shows the blue glow resulting from a beam of relativistic electrons as they slow down. This deceleration produces synchrotron light out of the beam line of the National Synchrotron Light Source.
Research
Hypothesis:
- Synchrotron radiation is prevalent from many astronomical sources.
Control groups
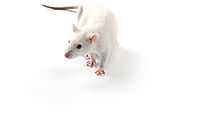
The findings demonstrate a statistically systematic change from the status quo or the control group.
“In the design of experiments, treatments [or special properties or characteristics] are applied to [or observed in] experimental units in the treatment group(s).[26] In comparative experiments, members of the complementary group, the control group, receive either no treatment or a standard treatment.[27]"[28]
Proof of concept
Def. a “short and/or incomplete realization of a certain method or idea to demonstrate its feasibility"[29] is called a proof of concept.
Def. evidence that demonstrates that a concept is possible is called proof of concept.
The proof-of-concept structure consists of
- background,
- procedures,
- findings, and
- interpretation.[30]
See also
References
- ↑ Uwe Arp (June 2003). "Synchrotron Radiation". Gaithersburg, Maryland USA: National Institute of Standards and Technology NIST). Retrieved 2014-04-02.
- ↑ Garcia, Leonard N. "The Jovian Decametric Radio Emission". NASA. Retrieved 2007-02-18.
- 1 2 "Jupiter, In: Wikipedia". San Francisco, California: Wikimedia Foundation, Inc. September 17, 2012. Retrieved 2012-09-27.
- ↑ Linda T. Elkins-Tanton (2006). Jupiter and Saturn. New York: Chelsea House. ISBN 0-8160-5196-8.
- ↑ Klein, M. J.; Gulkis, S.; Bolton, S. J. (1996). "Jupiter's Synchrotron Radiation: Observed Variations Before, During and After the Impacts of Comet SL9". NASA. Retrieved 2007-02-18.
- ↑ "synchrotron radiation, In: Wiktionary". San Francisco, California: Wikimedia Foundation, Inc. November 10, 2012. Retrieved 2012-12-21.
- ↑ L. Maraschi, G. Ghisellini and A. Celotti (September 1992). "A jet model for the gamma-ray emitting blazar 3C 279". The Astrophysical Journal 397 (1): L5-9. doi:10.1086/186531. http://adsabs.harvard.edu/abs/1992ApJ...397L...5M. Retrieved 2014-01-10.
- 1 2 Bill Keel (October 2003). "Jets, Superluminal Motion, and Gamma-Ray Bursts". Tucson, Arizona USA: University of Arizona. Retrieved 2014-03-19.
- 1 2 3 Morrison P (1967). "Extrasolar X-ray Sources". Ann Rev Astron Astrophys 5 (1): 325. doi:10.1146/annurev.aa.05.090167.001545.
- 1 2 Marshallsumter (April 15, 2013). X-ray astronomy, In: Wikipedia. San Francisco, California: Wikimedia Foundation, Inc. http://en.wikipedia.org/wiki/X-ray_astronomy. Retrieved 2013-05-11.
- ↑ "Active galactic nucleus, In: Wikipedia". San Francisco, California: Wikimedia Foundation, Inc. April 29, 2013. Retrieved 2013-05-06.
- ↑ Iosif Shklovskii (1953). "On the Nature of the Crab Nebula’s Optical Emission". Doklady Akademii Nauk SSSR 90: 983.
- ↑ B. J. Burn (1973). "A synchrotron model for the continuum spectrum of the Crab Nebula". Monthly Notices of the Royal Astronomical Society 165: 421.
- 1 2 G. Trottet, Säm Krucker, T. Lüthi, and A. Magun (May 1 2008). "Radio Submillimeter and γ-Ray Observations of the 2003 October 28 Solar Flare". The Astrophysical Journal 678 (1): 509. doi:10.1086/528787. http://iopscience.iop.org/0004-637X/678/1/509. Retrieved 2013-10-22.
- ↑ Synchrotron Radiation Damping in the LHC 2005 Joachim Tuckmantel.
- ↑ "Synchrotron radiation, In: Wikipedia". San Francisco, California: Wikimedia Foundation, Inc. August 25, 2012. Retrieved 2012-08-31.
- 1 2 3 4 I.A. Smith, R.P.J. Tilanus, J. van Paradijs, T.J. Galama, P.J. Groot, P. Vreeswijk, C. Kouveliotou, R.A.M. Wijers, and N. Tanvir (July 1999). "SCUBA sub-millimeter observations of gamma-ray bursters I. GRB 970508, 971214, 980326, 980329, 980519, 980703, 981220, 981226". Astronomy and Astrophysics 347 (07): 92-8. http://adsabs.harvard.edu/full/1999A%26A...347...92S. Retrieved 2013-10-22.
- ↑ "NGC 383, In: Wikipedia". San Francisco, California: Wikimedia Foundation, Inc. 22 February 2013. Retrieved 2014-07-18.
- ↑ Cox, A. N., ed (2000). Allen's Astrophysical Quantities. New York: Springer-Verlag. p. 124. ISBN 0-387-98746-0. http://books.google.com/?id=w8PK2XFLLH8C&pg=PA124.
- ↑ "Astronomy, In: Wikipedia". San Francisco, California: Wikimedia Foundation, Inc. August 28, 2012. Retrieved 2012-08-29.
- ↑ "Birkeland current, In: Wikipedia". San Francisco, California: Wikimedia Foundation, Inc. October 15, 2012. Retrieved 2012-11-17.
- ↑ S.G. Djorgovski et al. "A Tour of the Radio Universe". National Radio Astronomy Observatory. Retrieved 2014-03-16.
- ↑ Meisenheimer K, Röser H-J, Hiltner PR, Yates MG, Longair MS, Chini R, Perley RA; Roser; Hiltner; Yates; Longair; Chini; Perley (1989). "The synchrotron spectra of radio hotspots". Astronomy and Astrophysics 219: 63–86.
- ↑ "Radio galaxy, In: Wikipedia". San Francisco, California: Wikimedia Foundation, Inc. August 24, 2013. Retrieved 2013-10-04.
- 1 2 "Synchrotron function, In: Wikipedia". San Francisco, California: Wikimedia Foundation, Inc. December 5, 2011. Retrieved 2012-05-14.
- ↑ Klaus Hinkelmann, Oscar Kempthorne (2008). Design and Analysis of Experiments, Volume I: Introduction to Experimental Design (2nd ed.). Wiley. ISBN 978-0-471-72756-9. http://books.google.com/?id=T3wWj2kVYZgC&printsec=frontcover.
- ↑ R. A. Bailey (2008). Design of comparative experiments. Cambridge University Press. ISBN 978-0-521-68357-9. http://www.cambridge.org/uk/catalogue/catalogue.asp?isbn=9780521683579.
- ↑ "Treatment and control groups, In: Wikipedia". San Francisco, California: Wikimedia Foundation, Inc. May 18, 2012. Retrieved 2012-05-31.
- ↑ "proof of concept, In: Wiktionary". San Francisco, California: Wikimedia Foundation, Inc. November 10, 2012. Retrieved 2013-01-13.
- ↑ Ginger Lehrman and Ian B Hogue, Sarah Palmer, Cheryl Jennings, Celsa A Spina, Ann Wiegand, Alan L Landay, Robert W Coombs, Douglas D Richman, John W Mellors, John M Coffin, Ronald J Bosch, David M Margolis (August 13, 2005). "Depletion of latent HIV-1 infection in vivo: a proof-of-concept study". Lancet 366 (9485): 549-55. doi:10.1016/S0140-6736(05)67098-5. http://www.ncbi.nlm.nih.gov/pmc/articles/PMC1894952/. Retrieved 2012-05-09.
Further reading
- Uwe Arp (June 2003). "Synchrotron Radiation". Gaithersburg, Maryland USA: National Institute of Standards and Technology NIST). Retrieved 2014-04-02.
External links
- African Journals Online
- Bing Advanced search
- Google Books
- Google scholar Advanced Scholar Search
- International Astronomical Union
- JSTOR
- Lycos search
- NASA/IPAC Extragalactic Database - NED
- NASA's National Space Science Data Center
- NCBI All Databases Search
- Office of Scientific & Technical Information
- Questia - The Online Library of Books and Journals
- SAGE journals online
- The SAO/NASA Astrophysics Data System
- Scirus for scientific information only advanced search
- SDSS Quick Look tool: SkyServer
- SIMBAD Astronomical Database
- SIMBAD Web interface, Harvard alternate
- Spacecraft Query at NASA.
- SpringerLink
- Taylor & Francis Online
- WikiDoc The Living Textbook of Medicine
- Wiley Online Library Advanced Search
- Yahoo Advanced Web Search
|
|
This is a research project at http://en.wikiversity.org
![]() |
Development status: this resource is experimental in nature. |
![]() |
Educational level: this is a research resource. |
![]() |
Resource type: this resource is an article. |
![]() |
Resource type: this resource contains a lecture or lecture notes. |
![]() |
Subject classification: this is an astrophysics resource. |