Radiation astronomy/Subatomics
< Radiation astronomy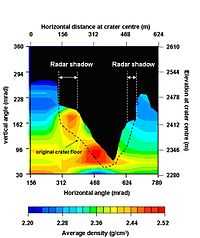
Subatomic astronomy is an observational astronomy using one or more subatomic particles or radiation.
A variety of subatomic particle astronomies have already been developed. These are highlighted below.
Potential particle astronomies are examined for their likelihood of becoming a successful astronomy.
Theoretical subatomic astronomy
The bare nuclei of atoms may qualify as a form of subatomic astronomy.
Def.
- "particles that are constituents of the atom, or are smaller than an atom; such as proton, neutron, electron, etc"[1] or
- "any length or mass that is smaller in scale than a the diameter of a hydrogen atom"[1]
are called subatomics, or subatomic, respectively.
As a bare uranium nucleus is smaller than a hydrogen atom in diameter, but much larger in mass, it qualifies as one of the subatomics. Here, subatomic is used to mean smaller than the diameter of a hydrogen atom.
A neutron star is one nucleus surrounded by an electron cloud. But, it is much larger than a hydrogen atom in diameter.
Cosmic-rays
“About 89% of cosmic rays are simple protons or hydrogen nuclei, 10% are helium nuclei or alpha particles, and 1% are the nuclei of heavier elements. ... Solitary electrons ... constitute much of the remaining 1%.”[2]
In cosmic-ray astronomy, cosmic rays are not charge balanced; that is, positive ions heavily outnumber electrons.
Lithium nuclei
The "evidence for the overwhelming majority of the Li-atoms in photospheres has its origin not only in nuclear synthesis near the stellar centers, but also by active processes in stellar atmospheres. [...] the lithium [resonance] line [is] near 478 keV."[3]
"Approximately 90% of lithium atoms originate from α - α reactions for the typical spectra of an accelerated particles on the Sun [...] During impulsive flares, interaction between the accelerated particles and the ambient medium occurs mainly at low altitudes, i.e., close to the footprints of loops."[3]
Alpha particles
“About 89% of cosmic rays are simple protons or hydrogen nuclei, 10% are helium nuclei or alpha particles, and 1% are the nuclei of heavier elements. ... Solitary electrons ... constitute much of the remaining 1%.”[2]
Helions
Def. a "nucleus of a helium-3 atom"[4] is called a helion.
Tritons
Energetic deuterons and tritons have been detected in solar flares.[5]
Deuterons
"The flux [of deuterons in cosmic rays at a geomagnetic latitude of 7.6°N] is found to be 4 ± 1.3 M-2 sec-1 sterad-1".[6]
Baryons
In "dense nuclear matter, such as neutron stars [it] has recently been discovered that kaon condensation in nuclear matter at a density of a few times normal nuclear matter may significantly reduce the upper mass limit of neutron stars [...] This clearly has an impact on astronomical observations. By exploiting the electron fermi level, we are able to predict kaon production at reasonable baryon number densities [...] Experimental detection of [dibaryons, hyperons] is a subtle matter [...] there is strong theoretical evidence that such states [as the dibaryon] do exist in nature. [...] the lightest dibaryon [...] is energetically stable against strong decay to [ΛΛ baryons] by 88 MeV. [The H dibaryon] is bound by 250 MeV."[7]
Neutrons
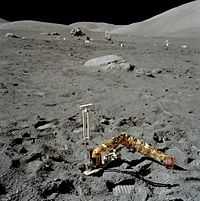
Neutron astronomy deals with the study of astronomical neutron sources (such as stars, planets, comets, nebulae, star clusters and galaxies and phenomena that originate outside the Earth's atmosphere, such as cosmic rays.
Protons
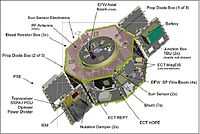
Proton astronomy per se often consists of directly or indirectly detecting the protons and deconvoluting a spatial, temporal, and spectral distribution.
"Proton astronomy should be possible; it may also provide indirect information on inter-galactic magnetic fields."[8]
"The Relativistic Proton Spectrometer (RPS) [measures] inner radiation belt protons with energies from 50 MeV-2 GeV. Such protons are known to pose a number of hazards to humans and spacecraft, including total ionizing dose, displacement damage, single event effects, and nuclear activation. The objectives of the investigation are to: (1) support the development of a new AP9/AE9 standard radiation model for spacecraft design; (2) to develop and test the model for RBSP data in general and RPS specifically; and, (3) to provide standardized worst-case specifications for dose rate, internal and deep dielectric chargins, and surface charging."[9]
Mesons
"[M]esons [...] are hadronic subatomic particles [...], bound together by the strong interaction. Because mesons are composed of sub-particles, they have a physical size, with a radius roughly one femtometre, which is about 2⁄3 the size of a proton or neutron."[10]
"Charged mesons decay (sometimes through intermediate particles) to form electrons and neutrinos. Uncharged mesons may decay to photons."[10]
"Mesons are not produced by radioactive decay, but appear in nature only as short-lived products of very high-energy interactions in matter ... In cosmic ray interactions, for example, such particles are ordinary protons and neutrons. Mesons are also frequently produced artificially in high-energy particle accelerators that collide protons, anti-protons, or other particles."[10]
Mesons are subject to "both the weak and strong interactions. Mesons with net electric charge also participate in the electromagnetic interaction."[10]
"While no meson is stable, those of lower mass are nonetheless more stable than the most massive mesons, and are easier to observe and study in particle accelerators or in cosmic ray experiments. They are also typically less massive than baryons, meaning that they are more easily produced in experiments, and thus exhibit certain higher energy phenomena more readily than baryons composed of the same quarks would."[10]
Potential mesons to be detected astronomically include: π, ρ, η, η′, φ, ω, J/ψ, ϒ, θ, K, B, D, and T.
B mesons
"The K0-K0 bar, D0-D0 bar, and B0-B0 bar oscillations are extremely sensitive to the K0 and K0 bar energy at rest. The energy is determined by the values mc2 with the related mass as well as the energy of the gravitational interaction. Assuming the CPT theorem for the inertial masses and estimating the gravitational potential through the dominant contribution of the gravitational potential of our Galaxy center, we obtain from the experimental data on the K0-K0 bar oscillations the following constraint: |(mg/mi)K0 - (mg/mi)K0 bar| ≤ 8·10-13, CL=90%. This estimation is model dependent and in particular it depends on a way we estimate the gravitational potential. Examining the K0-K0 bar, B0-B0 bar, and D0-D0 bar oscillations provides us also with weaker, but model independent constraints, which in particular rule out the very possibility of antigravity for antimatter."[11]
Upsilon mesons
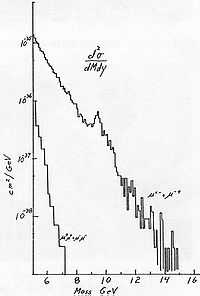
The plot on the right shows a peak at about 9.5 GeV due to the Upsilon meson.
Psions
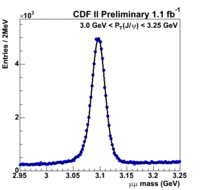
On the right is a graph of the production of psions at Fermilab.
Omega mesons
Omega meson production:[12]
Phi mesons
The phi meson (1020) has a mass of 1019.445 MeV. It decays per[13]
Rho mesons
Rho mesons occur in three states: ρ+, ρ-, and ρ0.[13] The rest masses are apparently the same at 775.4±0.4 and 775.49±0.34.[13] Decay products are π± + π0 or π+ + π-, respectively.[13]
Eta mesons
Eta mesons (547.863 ± 0.018 MeV) have the decay schemes:[12]
- η :
- η :
- η :
Eta prime mesons (957.78 ± 0.06 MeV) have the decay schemes:[12]
- η' :
- η' :
The charmed eta meson ηC(1S) has a rest mass of 2983.6 ± 0.7 MeV.[12]
D mesons
Kaons
"The muons created through decays of secondary pions and kaons are fully polarized, which results in electron/positron decay asymmetry, which in turn causes a difference in their production spectra."[15]
The "highest energy neutrinos from GRBs mainly come from kaons."[16]
Pions
"The Gamma-Ray Spectrometer (GRS) on [Solar Maximum Mission] SMM has detected [...] at least two of the flares have spectral properties >40 MeV that require gamma rays from the decay of neutral pions. [Pion] production can occur early in the impulsive phase as defined by hard X-rays near 100 keV."[17]
Tauons
"For ultrahigh energies the neutrino spectrum at the detector is influenced by neutrino-nucleon interactions and tauon decays during the passage through the interior of the earth."[18]
Muons
"TeV muons from γ ray primaries ... are rare because they are only produced by higher energy γ rays whose flux is suppressed by the decreasing flux at the source and by absorption on interstellar light."[8]
"[T]here is a window of opportunity for muon astronomy with the AMANDA, Lake Baikal, and MILAGRO detectors."[8]
Beta particles
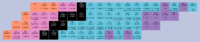
Credit: original: National Nuclear Data Center, stitched: Neokortex, cropped: Limulus.
"Beta particles are high-energy, high-speed electrons or positrons emitted by certain types of radioactive nuclei such as potassium-40. The beta particles emitted are a form of ionizing radiation also known as beta rays. The production of beta particles is termed beta decay. They are designated by the Greek letter beta (β)."[19]
At right is a graph or block diagram that shows the boundaries for nuclear particle stability. "[T]he boundaries ... are conceptualized as drip lines. The nuclear landscape is understood by plotting boxes, each of which represents a unique nuclear species, on a graph with the number of neutrons increasing on the abscissa and number of protons increasing along the ordinate, which is commonly referred to as the table of nuclides, being to nuclear physics what the more commonly known periodic table of the elements is to chemistry. However, an arbitrary combination of protons and neutrons does not necessarily yield a stable nucleus, and ultimately when continuing to add more of the same type of nucleons to a given nucleus, the newly formed nucleus will essentially undergo immediate decay where a nucleon of the same isospin quantum number (proton or neutron) is emitted; colloquially the nucleon has 'leaked' or 'dripped' out of the target nucleus, hence giving rise to the term "drip line". The nucleons drip out of such unstable nuclei for the same reason that water drips from a leaking faucet: the droplet, or nucleon in this case, sees a lower potential which is great enough to overcome surface tension in the case of water droplets, and the strong nuclear force in the case of proton emission or alpha decay. As nucleons are quantized, then only integer values are plotted on the table of isotopes, indicating that the drip line is not linear but instead looks like a step function up close."[20]
"Beta particles (electrons) are more penetrating [than alpha particles], but still can be absorbed by a few millimeters of aluminum. However, in cases where high energy beta particles are emitted shielding must be accomplished with low density materials, e.g. plastic, wood, water or acrylic glass (Plexiglas, Lucite). This is to reduce generation of Bremsstrahlung X-rays. In the case of beta+ radiation (positrons), the gamma radiation from the electron-positron annihilation reaction poses additional concern."[21]
As an example, "[t]he power into the Crab Nebula is apparently supplied by an outflow [wind] of ~1038 erg/s from the pulsar"[22] where there are "electrons (and positrons) in such a wind"[22]. These beta particles coming out of the pulsar are moving very close to light speed.
Electrons
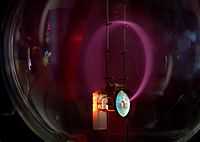
Although electron astronomy is usually not recognized as a formal branch of astronomy, the measurement of electron fluxes helps to understand a variety of natural phenomena.
""[E]lectron astronomy" has an interesting future".[23]
"Electron beams can be generated by thermionic emission, field emission or the anodic arc method. The generated electron beam is accelerated to a high kinetic energy and focused towards the [target]. When the accelerating voltage is between 20 kV – 25 kV and the beam current is a few amperes, 85% of the kinetic energy of the electrons is converted into thermal energy as the beam bombards the surface of the [target]. The surface temperature of the [target] increases resulting in the formation of a liquid melt. Although some of incident electron energy is lost in the excitation of X-rays and secondary emission, the [target] material evaporates under vacuum."[24]
Positrons
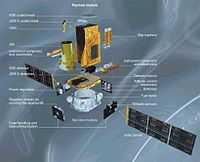
"Positron astronomy is 30 years old but remains in its infancy."[25]
"In 2009, [the] Fermi Gamma Ray Telescope in Earth orbit observed [an] intense burst of gamma rays corresponding to positron annihilations coming out of a storm formation. Scientists wouldn't have been surprised to see a few positrons accompanying any intense gamma ray burst, but the lightning flash detected by Fermi appeared to have produced about 100 trillion positrons. This has been reported by media in January 2011, it is an effect, never considered to happen before.[26]"[27]
Neutrinos
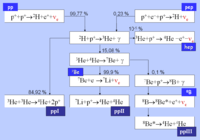
"The highest flux of solar neutrinos come directly from the proton-proton interaction, and have a low energy, up to 400 keV. There are also several other significant production mechanisms, with energies up to 18 MeV. [28]"[29]
Research
Hypothesis:
- Each extant subatomic particle may help to understand a radiation source.
Control groups
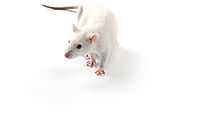
The findings demonstrate a statistically systematic change from the status quo or the control group.
“In the design of experiments, treatments [or special properties or characteristics] are applied to [or observed in] experimental units in the treatment group(s).[30] In comparative experiments, members of the complementary group, the control group, receive either no treatment or a standard treatment.[31]"[32]
Proof of concept
Def. a “short and/or incomplete realization of a certain method or idea to demonstrate its feasibility"[33] is called a proof of concept.
Def. evidence that demonstrates that a concept is possible is called proof of concept.
The proof-of-concept structure consists of
- background,
- procedures,
- findings, and
- interpretation.[34]
See also
References
- 1 2 "subatomic, In: Wiktionary". San Francisco, California: Wikimedia Foundation, Inc. 17 December 2014. Retrieved 2015-02-13.
- 1 2 "Cosmic ray, In: Wikipedia". San Francisco, California: Wikimedia Foundation, Inc. August 17, 2012. Retrieved 2012-08-17.
- 1 2 M. A. Livshits (July 1997). "The Amount of Lithium Produced during Impulsive Flares". Solar Physics 173 (2): 377-81. doi:10.1023/A:1004958522216. http://link.springer.com/article/10.1023/A:1004958522216#page-1. Retrieved 2014-10-01.
- ↑ "helion, In: Wiktionary". San Francisco, California: Wikimedia Foundation, Inc. 3 November 2013. Retrieved 2014-10-01.
- ↑ P. S. Freier and C. J. Waddington (1963). Energetic Deuterons and Tritons produced by Solar Flares, In: Solar Particles and Sun-Earth Relations. 1. pp. 139. Bibcode: 1963ICRC....1..139F. http://adsabs.harvard.edu//abs/1963ICRC....1..139F. Retrieved 2014-10-01.
- ↑ K. M. V. Apparao (1973). Flux of Cosmic Ray Deuterons with Rigidity Above 16.8 GV, In: Proceedings of the 13th International Conference on Cosmic Rays. 1. pp. 126-9. Bibcode: 1973ICRC....1..126A. http://adsabs.harvard.edu/full/1973ICRC....1..126A. Retrieved 2014-09-30.
- ↑ Karl Michael Westerberg (1996). "Hyperon Calculations in the Skyrme Model". Dissertation Abstracts International 57-04 (B): 2542. http://adsabs.harvard.edu/abs/1996PhDT.........2W. Retrieved 2014-10-03.
- 1 2 3 Francis Halzen and Dan Hooper (July 2002). "High-energy neutrino astronomy: the cosmic ray connection". Reports on Progress in Physics 65 (7): 1025-78. doi:10.1088/0034-4885/65/7/201. http://iopscience.iop.org/0034-4885/65/7/201. Retrieved 2011-11-24.
- ↑ Edwin V. Bell, II (August 16, 2013). "Van Allen Probe A (RBSP-A)". Washington, DC USA: National Space Science Data Center, NASA. Retrieved 2014-01-07.
- 1 2 3 4 5 "Meson, In: Wikipedia". San Francisco, California: Wikimedia Foundation, Inc. January 17, 2014. Retrieved 2014-01-20.
- ↑ Savely G. Karshenboim (2009). "Oscillations of neutral mesons and the equivalence principle for particles and antiparticles". Pis'ma v Zhurnal 'Fizika Ehlementarnykh Chastits i Atomnogo Yadra' 6 (155): 745-53. https://inis.iaea.org/search/search.aspx?orig_q=RN:41133347. Retrieved 2014-10-02.
- 1 2 3 4 K.A. Olive et al. (Particle Data Group) (2014). Chinese Physics C38: 090001. http://pdg.lbl.gov/2014/listings/rpp2014-list-omega-782.pdf. Retrieved 2015-02-11.
- 1 2 3 4 C. Amsler et al. (2008). "Particle listings".
- ↑ K. Kodama, N. Ushida1, C. Andreopoulos, N. Saoulidou, G. Tzanakos, P. Yager, B. Baller, D. Boehnlein, W. Freeman, B. Lundberg, J. Morfin, R. Rameika, J.C. Yun, J.S. Song, C.S. Yoon, S.H.Chung, P. Berghaus, M. Kubanstev, N.W. Reay, R. Sidwell, N. Stanton, S. Yoshida, S. Aoki, T. Hara, J.T. Rhee, D. Ciampa, C. Erickson, M. Graham, K. Heller, R. Rusack, R. Schwienhorst, J. Sielaff, J. Trammell, J. Wilcox, K. Hoshino, H. Jiko, M. Miyanishi, M. Komatsu, M. Nakamura, T. Nakano, K. Niwa, N. Nonaka, K. Okada, O. Sato, T. Akdogan, V. Paolone, C. Rosenfeld, A. Kulik, T. Kafka, W. Oliver, T. Patzak, and J. Schneps (April 12, 2001). "Observation of tau neutrino interactions". Physics Letters B 504 (3): 218-24. http://www.sciencedirect.com/science/article/pii/S0370269301003070. Retrieved 2014-03-10.
- ↑ I. V. Moskalenko and A. W. Strong (February 1, 1998). "Production and propagation of cosmic-ray positrons and electrons". The Astrophysical Journal 493 (2): 694-707. doi:10.1086/305152. http://iopscience.iop.org/0004-637X/493/2/694. Retrieved 2014-02-01.
- ↑ K. Asano and S. Nagataki (20 March 2006). "Very High Energy Neutrinos Originating from Kaons in Gamma-Ray Bursts". The Astrophysical Journal Letters 640 (1): L9. doi:10.1086/503291. http://arxiv.org/pdf/astro-ph/0603107.pdf. Retrieved 2014-10-02.
- ↑ Forrest, D. J., Vestrand, W. T., Chupp, E. L., Rieger, E., Cooper, J. F., & Share, G. H. (August 1985). Neutral Pion Production in Solar Flares, In: 19th International Cosmic Ray Conference. 4. NASA. pp. 146-9. Bibcode: 1985ICRC....4..146F. http://adsabs.harvard.edu/full/1985ICRC....4..146F. Retrieved 2014-10-01.
- ↑ Hettlage, C.; Mannheim, K. (20-25 September 1999). "Tau Sources in the Sky". AG Abstract Services 15 (04). http://adsabs.harvard.edu//abs/1999AGM....15..I04H. Retrieved 2014-10-02.
- ↑ "Beta particle, In: Wikipedia". San Francisco, California: Wikimedia Foundation, Inc. August 22, 2012. Retrieved 2012-08-31.
- ↑ "Nuclear drip line, In: Wikipedia". San Francisco, California: Wikimedia Foundation, Inc. July 18, 2012. Retrieved 2012-08-31.
- ↑ "Radiation, In: Wikipedia". San Francisco, California: Wikimedia Foundation, Inc. May 31, 2012. Retrieved 2012-06-02.
- 1 2 D. B. Wilson and M. J. Rees (October 1978). "Induced Compton scattering in pulsar winds". Monthly Notices of the Royal Astronomical Society 185 (10): 297-304.
- ↑ H. S. Hudson and A. B. Galvin (September 1997). A. Wilson. ed. Correlated Studies at Activity Maximum: the Sun and the Solar Wind, In: Correlated Phenomena at the Sun, in the Heliosphere and in Geospace. Noordwijk, The Netherlands: European Space Agency. pp. 275-82. ISBN 92-9092-660-0.
- ↑ Dgray (January 17, 2008). "Materials Science and Engineering/Doctoral review questions/Daily Discussion Topics/01162008, In: Wikiversity". Retrieved 2013-07-21.
- ↑ P.A.Milne, J.D.Kurfess, R.L.Kinzer, M.D.Leising, D.D.Dixon (April 2000). Investigations of positron annihilation radiation, In: Proceedings of the 5th COMPTON Symposium. 510. Washington, DC: American Institute of Physics. pp. 21-30. doi:10.1063/1.1303167. http://arxiv.org/abs/astro-ph/9911184. Retrieved 2011-11-25.
- ↑ http://news.nationalgeographic.com/news/2011/01/110111-thunderstorms-antimatter-beams-fermi-radiation-science-space/
- ↑ "Lightning, In: Wikipedia". San Francisco, California: Wikimedia Foundation, Inc. May 7, 2013. Retrieved 2013-05-07.
- ↑ A. Bellerive, Review of solar neutrino experiments. Int.J.Mod.Phys. A19 (2004) 1167-1179
- ↑ "Solar neutrino, In: Wikipedia". San Francisco, California: Wikimedia Foundation, Inc. April 4, 2012. Retrieved 2012-11-08.
- ↑ Klaus Hinkelmann, Oscar Kempthorne (2008). Design and Analysis of Experiments, Volume I: Introduction to Experimental Design (2nd ed.). Wiley. ISBN 978-0-471-72756-9. http://books.google.com/?id=T3wWj2kVYZgC&printsec=frontcover.
- ↑ R. A. Bailey (2008). Design of comparative experiments. Cambridge University Press. ISBN 978-0-521-68357-9. http://www.cambridge.org/uk/catalogue/catalogue.asp?isbn=9780521683579.
- ↑ "Treatment and control groups, In: Wikipedia". San Francisco, California: Wikimedia Foundation, Inc. May 18, 2012. Retrieved 2012-05-31.
- ↑ "proof of concept, In: Wiktionary". San Francisco, California: Wikimedia Foundation, Inc. November 10, 2012. Retrieved 2013-01-13.
- ↑ Ginger Lehrman and Ian B Hogue, Sarah Palmer, Cheryl Jennings, Celsa A Spina, Ann Wiegand, Alan L Landay, Robert W Coombs, Douglas D Richman, John W Mellors, John M Coffin, Ronald J Bosch, David M Margolis (August 13, 2005). "Depletion of latent HIV-1 infection in vivo: a proof-of-concept study". Lancet 366 (9485): 549-55. doi:10.1016/S0140-6736(05)67098-5. http://www.ncbi.nlm.nih.gov/pmc/articles/PMC1894952/. Retrieved 2012-05-09.
External links
This is a research project at http://en.wikiversity.org
![]() |
Development status: this resource is experimental in nature. |
![]() |
Educational level: this is a research resource. |
![]() |
Resource type: this resource is an article. |
![]() |
Resource type: this resource contains a lecture or lecture notes. |
![]() |
Subject classification: this is an astronomy resource. |