Radiation astronomy/Detectors
< Radiation astronomy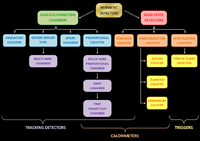
Radiation detectors provide a signal that is converted to an electric current. The device is designed so that the current provided is proportional to the characteristics of the incident radiation.
There are detectors that provide a change in substance as the signal and these may be automated to provide an electric current or quantified proportional to the amount of new substance.
Astronomy
A detector in radiation astronomy may need to be able to separate a collection of incoming radiation to obtain a clear set of signals for the radiation of interest. For example, a detector designed for red astronomy may need to be on the rocky-object surface of the Earth to separate X-rays and gamma-rays from red rays.
Radiation
Def. an action or process of throwing or sending out a traveling ray in a line, beam, or stream of small cross section is called radiation.
"Radiation may affect materials and devices in deleterious ways:"[1]
- By causing the materials to become radioactive (mainly by neutron activation, or in presence of high-energy gamma radiation by photodisintegration).
- By nuclear transmutation of the elements within the material including, for example, the production of Hydrogen and Helium which can in turn alter the mechanical properties of the materials and cause swelling and embrittlement.
- By radiolysis (breaking chemical bonds) within the material, which can weaken it, cause it to swell, polymerize, promote corrosion, cause belittlements, promote cracking or otherwise change its desirable mechanical, optical, or electronic properties.
- By formation of reactive compounds, affecting other materials (e.g. ozone cracking by ozone formed by ionization of air).
- By ionization, causing electrical breakdown, particularly in semiconductors employed in electronic equipment, with subsequent currents introducing operation errors or even permanently damaging the devices.
"Devices intended for high radiation environments such as the nuclear industry and extra atmospheric (space) applications may be made radiation hard to resist such effects through design, material selection, and fabrication methods."[1]
"Environments with high levels of ionizing radiation create special design challenges. A single charged particle can knock thousands of electrons loose, causing electronic noise and signal spikes. In the case of digital circuits, this can cause results which are inaccurate or unintelligible. This is a particularly serious problem in the design of artificial satellites, spacecraft, military aircraft, nuclear power stations, and nuclear weapons. In order to ensure the proper operation of such systems, manufacturers of integrated circuits and sensors intended for the military or aerospace markets employ various methods of radiation hardening. The resulting systems are said to be rad(iation)-hardened, rad-hard, or (within context) hardened."[2]
Planetary sciences
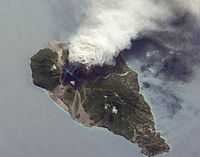
Oblique images such as the one at right are taken by astronauts looking out from the International Space Station (ISS) at an angle, rather than looking straight downward toward the Earth (a perspective called a nadir view), as is common with most remotely sensed data from satellites. An oblique view gives the scene a more three-dimension quality, and provides a look at the vertical structure of the volcanic plume. While much of the island is covered in green vegetation, grey deposits that include pyroclastic flows and volcanic mud-flows (lahars) are visible extending from the volcano toward the coastline. When compared to its extent in earlier views, the volcanic debris has filled in more of the eastern coastline. Urban areas are visible in the northern and western portions of the island; they are recognizable by linear street patterns and the presence of bright building rooftops. The silver-grey appearance of the Caribbean Sea surface is due to sun-glint, which is the mirror-like reflection of sunlight off the water surface back towards the hand-held camera on-board the ISS. The sun-glint highlights surface wave patterns around the island.
Theoretical radiation detectors
Def. "a device that recovers information of interest contained in a modulated wave"[3] is called a detector.
Def. “[a] device capable of registering a specific substance or physical phenomenon”[4] is called a detector.
Def. “a device used to detect, track, and/or identify high-energy particles, such as those produced by nuclear decay, cosmic radiation, or reactions in a particle accelerator”[5] is called a radiation detector.
Def. “[a] device or organ that detects certain external stimuli and responds in a distinctive manner”[6] is called a sensor.
"Humans have a multitude of senses. Sight (ophthalmoception), hearing (audioception), taste (gustaoception), smell (olfacoception or olfacception), and touch (tactioception) are the five traditionally recognized. While the ability to detect other stimuli beyond those governed by the traditional senses exists, including temperature (thermoception), kinesthetic sense (proprioception), pain (nociception), balance (equilibrioception), acceleration (kinesthesioception) ..., and various internal stimuli (e.g. the different chemoreceptors for detecting salt and carbon dioxide concentrations in the blood), ... some species [are] able to sense electrical and magnetic fields, and detect water pressure and currents."[7]
"Many sensors generate outputs that reflect the rate of change in attitude. These require a known initial attitude, or external information to use them to determine attitude. Many of this class of sensor have some noise, leading to inaccuracies if not corrected by absolute attitude sensors."[8]
"Gyroscopes are devices that sense rotation in three-dimensional space without reliance on the observation of external objects. Classically, a gyroscope consists of a spinning mass, but there are also "Laser Gyros" utilizing coherent light reflected around a closed path. Another type of "gyro" is a hemispherical resonator gyro where a crystal cup shaped like a wine glass can be driven into oscillation just as a wine glass "sings" as a finger is rubbed around its rim. The orientation of the oscillation is fixed in inertial space, so measuring the orientation of the oscillation relative to the spacecraft can be used to sense the motion of the spacecraft with respect to inertial space.[9]"[8]
"Motion Reference Units are single- or multi-axis motion sensors. They utilize Micro-Electro-Mechanical-Structure (MEMS) sensor technology. These sensors are revolutionizing inertial sensor technology by bringing together micro-electronics with micro-machining technology, to make complete systems-on-a-chip with high accuracy."[8]
"A horizon sensor is an optical instrument that detects light from the 'limb' of the Earth's atmosphere, i.e., at the horizon. Thermal Infrared sensing is often used, which senses the comparative warmth of the atmosphere, compared to the much colder cosmic background. This sensor provides orientation with respect to the earth about two orthogonal axes. It tends to be less precise than sensors based on stellar observation. Sometimes referred to as an Earth Sensor."[8]
"Similar to the way that a terrestrial gyrocompass uses a pendulum to sense local gravity and force its gyro into alignment with earth's spin vector, and therefore point north, an orbital gyrocompass uses a horizon sensor to sense the direction to earth's center, and a gyro to sense rotation about an axis normal to the orbit plane. Thus, the horizon sensor provides pitch and roll measurements, and the gyro provides yaw. See Tait-Bryan angles."[8]
"Gadolinium oxysulfide is a promising luminescent host material, because of its high density (7.32 g/cm3) and high effective atomic number of Gd. These characteristics lead to a high stopping power for X-ray radiation."[10]
Cadmium telluride (CdTe) "doped with chlorine is used as a radiation detector for [X-rays], gamma rays, beta particles and alpha particles. CdTe can operate at room temperature allowing the construction of compact detectors for a wide variety of applications in nuclear spectroscopy.[11] The properties that make CdTe superior for the realization of high performance gamma- and x-ray detectors are high atomic number, large bandgap and high electron mobility ~1100 cm2/V·s, which result in high intrinsic μτ (mobility-lifetime) product and therefore high degree of charge collection and excellent spectral resolution."[12]
Entities
Def. "the fraction of photoelectric events which end up in the photopeak of the measured energy spectrum"[13] is called the photopeak efficiency (ε).
Ending up in the photopeak means within ± 1 full-width at half maximum (FWHM) of the peak of the distribution.[13]
"The peak to valley ratio is commonly used as a token for ε."[13]
"Another common practice is to fit an exponential function to the “valley” and to extrapolate the fit to lower pulse heights to estimate the fraction of counts hidden in the Compton continuum."[13]
"We have used a calibrated Cs137 source to determine the absolute photopeak efficiency at 662 keV. The source was placed at a sufficiently large distance from the detector so that the event rate was low and the dead time was less than 20%. Based on a log-histogram of the time intervals between events, the dead-time has been estimated to a fractional accuracy of better than 5%. We determine the photopeak efficiency by comparing the dead-time corrected event rate in the photopeak with the theoretical expectation assuming a perfect detector."[13]
Def. "the average energy loss of the particle per unit path length"[14] is called the stopping power.
Def. "the slowing down of a projectile ion due to the inelastic collisions between bound electrons in the medium and the ion moving through it"[14] is called the electronic stopping power.
Def. "the elastic collisions between the projectile ion and atoms in the sample ... [involving] the interaction of the ion with the nuclei in the target"[14] is called the nuclear stopping power.
Radiation astronomy sources
"[T]he hardware setup also defines key experimental parameters, such as source-detector distance, solid angle and detector shielding."[15]
"Since the energy of a thermal neutron is relatively low, charged particle reaction is discrete (i.e., essentially monoenergetic) while other reactions such as gamma reactions will span a broad energy range, it is possible to discriminate among the sources."[15]
"The other sources of noise, such as alpha and beta particles, can be eliminated by various shielding materials, such as lead, plastic, thermo-coal, etc. Thus, photons cause major interference in neutron detection, since it is uncertain if neutrons or photons are being detected by the neutron detector."[15]
"A number of sources of X-ray photons have been used; these include X-ray generators, betatrons, and linear accelerators (linacs). For gamma rays, radioactive sources such as 192Ir, 60Co or 137Cs are used."[16]
"While in the past radium and radon have both been used for radiography, they have fallen out of use as they are radiotoxic alpha radiation emitters which are expensive; iridium-192 and cobalt-60 are far better photon sources."[16]
Objects
"Feature-based object recognizers generally work by pre-capturing a number of fixed views of the object to be recognized, extracting features from these views, and then in the recognition process, matching these features to the scene and enforcing geometric constraints."[17]
"Object recognition – in computer vision, this is the task of finding a given object in an image or video sequence. Humans recognize a multitude of objects in images with little effort, despite the fact that the image of the objects may vary somewhat in different view points, in many different sizes / scale or even when they are translated or rotated. Objects can even be recognized when they are partially obstructed from view. This task is still a challenge for computer vision systems in general."[18]
Continua
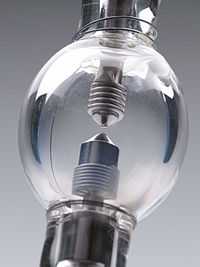
"A xenon arc lamp is a specialized type of gas discharge lamp, an electric light that produces light by passing electricity through ionized xenon gas at high pressure. It produces a bright white light that closely mimics natural sunlight."[19]
"[C]ontinuous spectra (as in bremsstrahlung and thermal radiation) are usually associated with free particles, such as atoms in a gas, electrons in an electron beam, or conduction band electrons in a metal. In particular, the position and momentum of a free particle have a continuous spectrum, but when the particle is confined to a limited space their spectra become discrete."[20]
"Inverse Compton scattering is important in astrophysics. In X-ray astronomy, the accretion disc surrounding a black hole is presumed to produce a thermal spectrum. The lower energy photons produced from this spectrum are scattered to higher energies by relativistic electrons in the surrounding corona."[21]
Emissions
"Condensed noble gases, most notably liquid xenon and liquid argon, are excellent radiation detection media. They can produce two signatures for each particle interaction: a fast flash of light (scintillation) and the local release of charge (ionisation). In two-phase xenon – so called since it involves liquid and gas phases in equilibrium – the scintillation light produced by an interaction in the liquid is detected directly with photomultiplier tubes; the ionisation electrons released at the interaction site are drifted up to the liquid surface under an external electric field, and subsequently emitted into a thin layer of xenon vapour. Once in the gas, they generate a second, larger pulse of light (electroluminescence or proportional scintillation), which is detected by the same array of photomultipliers. These systems are also known as xenon 'emission detectors'.[22]"[23]
Absorptions
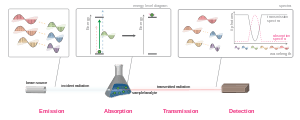
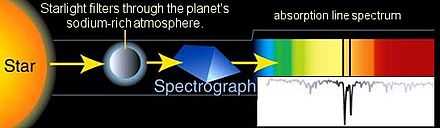
"Absorption spectroscopy refers to spectroscopic techniques that measure the absorption of radiation, as a function of frequency or wavelength, due to its interaction with a sample. The sample absorbs energy, i.e., photons, from the radiating field. The intensity of the absorption varies as a function of frequency, and this variation is the absorption spectrum. Absorption spectroscopy is performed across the electromagnetic spectrum."[24]
This example in the image at center discusses the general principle using visible light as a specific example. A white beam source – emitting light of multiple wavelengths – is focused on a sample (the complementary color pairs are indicated by the yellow dotted lines). Upon striking the sample, photons that match the energy gap of the molecules present (green light in this example) are absorbed in order to excite the molecule. Other photons transmit unaffected and, if the radiation is in the visible region (400-700nm), the sample color is the complementary color of the absorbed light. By comparing the attenuation of the transmitted light with the incident, an absorption spectrum can be obtained.
Bands
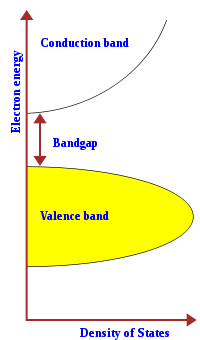
"[A] band gap, also called an energy gap or bandgap, is an energy range in a solid where no electron states can exist. In graphs of the electronic band structure of solids, the band gap generally refers to the energy difference (in electron volts) between the top of the valence band and the bottom of the conduction band in insulators and semiconductors. This is equivalent to the energy required to free an outer shell electron from its orbit about the nucleus to become a mobile charge carrier, able to move freely within the solid material. So the band gap is a major factor determining the electrical conductivity of a solid. Substances with large band gaps are generally insulators, those with smaller band gaps are semiconductors, while conductors either have very small band gaps or none, because the valence and conduction bands overlap."[25]
"Every solid has its own characteristic energy band structure. This variation in band structure is responsible for the wide range of electrical characteristics observed in various materials. In semiconductors and insulators, electrons are confined to a number of bands of energy, and forbidden from other regions. The term "band gap" refers to the energy difference between the top of the valence band and the bottom of the conduction band. Electrons are able to jump from one band to another. However, in order for an electron to jump from a valence band to a conduction band, it requires a specific minimum amount of energy for the transition. The required energy differs with different materials. Electrons can gain enough energy to jump to the conduction band by absorbing either a phonon (heat) or a photon (light)."[25]
"[T]he electronic band structure (or simply band structure) of a solid describes those ranges of energy, called energy bands, that an electron within the solid may have ("allowed bands"), and ranges of energy called band gaps ("forbidden bands"), which it may not have."[26]
Backgrounds
"The main components of background noise in neutron detection are high-energy photons, which aren't easily eliminated by physical barriers."[15]
"[N]oise is a random fluctuation in an electrical signal, a characteristic of all electronic circuits.[27] Noise generated by electronic devices varies greatly, as it can be produced by several different effects. Thermal noise is unavoidable at non-zero temperature (see fluctuation-dissipation theorem), while other types depend mostly on device type (such as shot noise,[27][28] which needs steep potential barrier) or manufacturing quality and semiconductor defects, such as conductance fluctuations, including 1/f noise."[29]
"[N]oise is an error or undesired random disturbance of a useful information signal, introduced before or after the detector and decoder. The noise is a summation of unwanted or disturbing energy from natural and sometimes man-made sources. Noise is, however, typically distinguished from interference, (e.g. cross-talk, deliberate jamming or other unwanted electromagnetic interference from specific transmitters), for example in the signal-to-noise ratio (SNR), signal-to-interference ratio (SIR) and signal-to-noise plus interference ratio (SNIR) measures. Noise is also typically distinguished from distortion, which is an unwanted alteration of the signal waveform, for example in the signal-to-noise and distortion ratio (SINAD). In a carrier-modulated passband analog communication system, a certain carrier-to-noise ratio (CNR) at the radio receiver input would result in a certain signal-to-noise ratio in the detected message signal. In a digital communications system, a certain Eb/N0 (normalized signal-to-noise ratio) would result in a certain bit error rate (BER)."[29]
"[S]pikes are fast, short duration electrical transients in voltage (voltage spikes), current (current spikes), or transferred energy (energy spikes) in an electrical circuit."[30]
"Fast, short duration electrical transients (overvoltages) in the electric potential of a circuit are typically caused by"[30]
- Lightning strikes,
- Power outages,
- Tripped circuit breakers,
- Short circuits,
- Power transitions in other large equipment on the same power line,
- Malfunctions caused by the power company,
- Electromagnetic pulses (EMP) with electromagnetic energy distributed typically up to the 100 kHz and 1 MHz frequency range, or
- Inductive spikes.
"For sensitive electronics, excessive current can flow if this voltage spike exceeds a material's breakdown voltage, or if it causes avalanche breakdown. In semiconductor junctions, excessive electric current may destroy or severely weaken that device. An avalanche diode, transient voltage suppression diode, transil, varistor, overvoltage crowbar, or a range of other overvoltage protective devices can divert (shunt) this transient current thereby minimizing voltage.[31]"[30]
Meteors
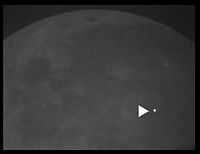
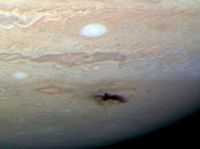
Usually, a meteor detector is designed for another form of radiation that the meteor may radiate.
In the image at right, a 0.3 m meteor has impacted a meteor detector, in this case the Moon, and created a scintillation event that in turn is detected by a photoelectronic detector system.
In the image at left, a meteor has impacted another detector, here Jupiter, but instead of a scintillation event has created a lowering of albedo as detected by the photoelectronic system, the Hubble Space Telescope.
Cosmic rays
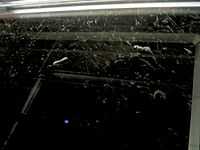
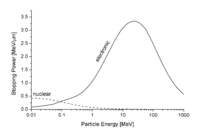
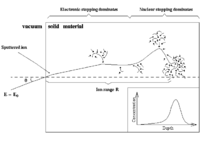
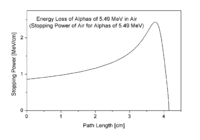
“The basic set-up consists of 1600 water tanks (water Cherenkov Detectors, similar to the Haverah Park experiment) distributed over 3,000 square kilometres (1,200 sq mi), along with four atmospheric fluorescence detectors (similar to the High Resolution Fly's Eye) overseeing the surface array.”[33]
“The Pierre Auger Observatory is unique in that it is the first experiment that combines both ground and fluorescence detectors at the same site thus allowing cross-calibration and reduction of systematic effects that may be peculiar to each technique. The Cherenkov detectors use three large photomultiplier tubes to detect the Cherenkov radiation produced by high-energy particles passing through water in the tank. The time of arrival of high-energy particles from the same shower at several tanks is used to calculate the direction of travel of the original particle. The fluorescence detectors are used to track the particle shower's glow on cloudless moonless nights, as it descends through the atmosphere.”[33]
"The cloud chamber, also known as the Wilson chamber, is a particle detector used for detecting ionizing radiation. In its most basic form, a cloud chamber is a sealed environment containing a supersaturated vapor of water or alcohol. When a charged particle (for example, an alpha or beta particle) interacts with the mixture, it ionizes it. The resulting ions act as condensation nuclei, around which a mist will form (because the mixture is on the point of condensation). The high energies of alpha and beta particles mean that a trail is left, due to many ions being produced along the path of the charged particle. These tracks have distinctive shapes (for example, an alpha particle's track is broad and shows more evidence of deflection by collisions, while an electron's is thinner and straight). When any uniform magnetic field is applied across the cloud chamber, positively and negatively charged particles will curve in opposite directions, according to the Lorentz force law with two particles of opposite charge"[34]
"The diffusion cloud chamber ... differs from the expansion cloud chamber in that it is continuously sensitized to radiation, and in that the bottom must be cooled to a rather low temperature, generally as cold as -15 degrees fahrenheit. Alcohol vapor is also often used due to its different phase transition temperatures. Dry-ice-cooled cloud chambers are a common demonstration and hobbyist device; the most common fluid used in them is isopropyl alcohol, though methyl alcohol can be encountered as well. There are also water-cooled diffusion cloud chambers, using ethylene glycol.[34]
"The bubble chamber ... reveals the tracks of subatomic particles ... as trails of bubbles in a superheated liquid, usually liquid hydrogen. Bubble chambers can be made physically larger than cloud chambers, and since they are filled with much-denser liquid material, they reveal the tracks of much more energetic particles."[34]
"The ... spark chamber is an electrical device that uses a grid of uninsulated electric wires in a chamber, with voltages applied between the wires. Microscopic charged particles cause some ionization of the air along the path of the particle, and this ionization causes sparks to fly between the associated wires. The presence and location of these sparks is then registered electrically, and the information is stored for later analysis, such as by a digital computer."[34]
"Cosmic radiation is a significant obstacle to a manned space flight to Mars. Accurate measurements of the cosmic ray environment are needed to plan appropriate countermeasures. Most cosmic ray studies are done by balloon-borne satellites with flight times that are measured in days; these studies have shown significant variations. AMS-02 [image in the superluminal detector section] will be operative on the ISS for a nominal mission of 3 years, gathering an immense amount of accurate data and allowing measurements of the long term variation of the cosmic ray flux over a wide energy range, for nuclei from protons to iron. After the nominal mission, AMS-02 can continue to provide cosmic ray measurements. In addition to the understanding the radiation protection required for manned interplanetary flight, this data will allow the interstellar propagation and origins of cosmic rays to be pinned down."[35]
The second figure on the right shows the electronic and nuclear stopping power of aluminum single crystal for aluminum ions. These stopping powers are versus particle energy per nucleon. The maximum of the nuclear stopping curve typically occurs at energies of the order of 1 keV per nucleon.
The third figure at right illustrates the slowing down of a single ion in a solid material.
"In the beginning of the slowing-down process at high energies, the ion is slowed down mainly by electronic stopping, and it moves almost in a straight path. When the ion has slowed down sufficiently, the collisions with nuclei (the nuclear stopping) become more and more probable, finally dominating the slowing down. When atoms of the solid receive significant recoil energies when struck by the ion, they will be removed from their lattice positions, and produce a cascade of further collisions in the material. These collision cascades are the main cause of damage production during ion implantation in metals and semiconductors."[14]
"When the energies of all atoms in the system have fallen below the threshold displacement energy, the production of new damage ceases, and the concept of nuclear stopping is no longer meaningful. The total amount of energy deposited by the nuclear collisions to atoms in the materials is called the nuclear deposited energy."[14]
"The inset in the figure shows a typical range distribution of ions deposited in the solid. The case shown here might for instance be the slowing down of a 1 MeV silicon ion in silicon. The mean range for a 1 MeV ion is typically in the micrometer range."[14]
Fourth right is an illustration of a Bragg curve. The stopping power and hence, the density of ionization, usually increases toward the end of range and reaches a maximum, the Bragg peak, shortly before the energy drops to zero.
Neutrons
“Detection hardware refers to the kind of neutron detector used [such as] the scintillation detector and to the electronics used in the detection setup. Further, the hardware setup also defines key experimental parameters, such as source-detector distance, solid angle and detector shielding. Detection software consists of analysis tools that perform tasks such as graphical analysis to measure the number and energies of neutrons striking the detector.”[36]
“Neutrons react with a number of materials through elastic scattering producing a recoiling nucleus, inelastic scattering producing an excited nucleus, or absorption with transmutation of the resulting nucleus. Most detection approaches rely on detecting the various reaction products.”[36]
“[D]etection approaches for neutrons fall into several major categories[37]:
- Absorptive reactions with prompt reactions - Low energy neutrons are typically detected indirectly through absorption reactions. Typical absorber materials used have high cross sections for absorption of neutrons and include Helium-3, Lithium-6, Boron-10, and Uranium-235. Each of these reacts by emission of high energy ionized particles, the ionization track of which can be detected by a number of means. Commonly used reactions include 3He(n,p) 3H, 6Li(n,α) 3H, 10B(n,α) 7Li and the fission of uranium.[37]
- Activation processes - Neutrons may be detected by reacting with absorbers in a radiative capture, spallation or similar reaction, producing reaction products which then decay at some later time, releasing beta particles or gammas. Selected materials (e.g., indium, gold, rhodium, iron (56Fe(n,p)56Mn), aluminum27Al(n,α)24Na), niobium (93Nb(n,2n)92mNb), & silicon (28Si(n,p)28Al)) have extremely large cross sections for the capture of neutrons within a very narrow band of energy. Use of multiple absorber samples allows characterization of the neutron energy spectrum. Activation also allows recreation of an historic neutron exposure (e.g., forensic recreation of neutron exposures during an accidental criticality).[37]
- Elastic scattering reactions (also referred to as proton-recoil) - High energy neutrons are typically detected indirectly through elastic scattering reactions. Neutron collide with the nucleus of atoms in the detector, transferring energy to that nucleus and creating an ion, which is detected. Since the maximum transfer of energy occurs when the mass of the atom with which the neutron collides is comparable to the neutron mass, hydrogenous [materials with a high hydrogen content such as water or plastic] materials are often the preferred medium for such detectors.[37]”[36]
Protons
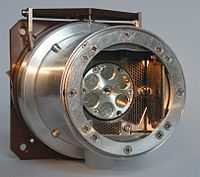
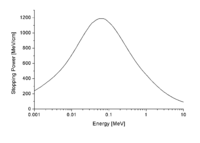
"Some of the alpha particles are absorbed by the atomic nuclei. The [alpha,proton] process produces protons of a defined energy which are detected. Sodium, magnesium, silicon, aluminium and sulfur can be detected by this method. This method was only used in the Mars Pathfinder APXS."[38]
At right, the second figure shows the stopping power of aluminum metal single crystal for protons.
"Choosing materials with the largest stopping powers enables thinner detectors to be produced with resulting benefits in radiation tolerance (which is a bulk effect) and lower leakage currents. Alternatively, choosing smaller stopping powers will increase scattering efficiency, which is a requirement for polarimetry, or say, the upper detection plane of a double Compton telescope."[39]
Electrons
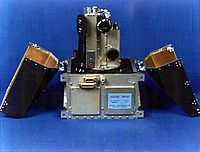
The Energetic Particles Detector (EPD) aboard the Galileo Orbiter is "designed to measure the numbers and energies of ... electrons whose energies exceed about 20 keV ... The EPD [can] also measure the direction of travel of [electrons] ... The EPD [uses] silicon solid state detectors and a time-of-flight detector system to measure changes in the energetic [electron] population at Jupiter as a function of position and time."[40]
"[The] two bi-directional, solid-state detector telescopes [are] mounted on a platform which [is] rotated by a stepper motor into one of eight positions. This rotation of the platform. combined with the spinning of the orbiter in a plane perpendicular to the platform rotation, [permits] a 4-pi [or 4π] steradian coverage of incoming [electrons]. The forward (0 degree) ends of the two telescopes [have] an unobstructed view over the [4π] sphere or [can] be positioned behind a shield which not only [prevents] the entrance of incoming radiation, but [contains] a source, thus allowing background corrections and in-flight calibrations to be made. ... The 0 degree end of the [Low-Energy Magnetospheric Measurements System] LEMMS [uses] magnetic deflection to separate incoming electrons and ions. The 180 degree end [uses] absorbers in combination with the detectors to provide measurements of higher-energy electrons ... The LEMMS [provides] measurements of electrons from 15 keV to greater than 11 MeV ... in 32 rate channels."[41]
Positrons
"In the first 18 months of operations, AMS-02 [image under Cherenkov detectors] recorded 6.8 million positron (an antimatter particle with the mass of an electron but a positive charge) and electron events produced from cosmic ray collisions with the interstellar medium in the energy range between 0.5 giga-electron volt (GeV) and 350 GeV. These events were used to determine the positron fraction, the ratio of positrons to the total number of electrons and positrons. Below 10 GeV, the positron fraction decreased with increasing energy, as expected. However, the positron fraction increased steadily from 10 GeV to 250 GeV. This increase, seen previously though less precisely by instruments such as the Payload for Matter/antimatter Exploration and Light-nuclei Astrophysics (PAMELA) and the Fermi Gamma-ray Space Telescope, conflicts with the predicted decrease of the positron fraction and indicates the existence of a currently unidentified source of positrons, such as pulsars or the annihilation of dark matter particles. Furthermore, researchers observed an unexpected decrease in slope from 20 GeV to 250 GeV. The measured positron to electron ratio is isotropic, the same in all directions."[35]
Muons
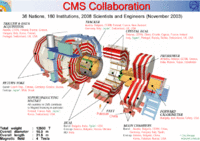
"With γ ray energy 50 times higher than the muon energy and a probability of muon production by the γ's of about 1%, muon detectors can match the detection efficiency of a GeV satellite detector if their effective area is larger by 104."[42]
Neutrinos
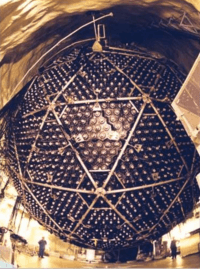
"A neutrino detector is ... designed to study neutrinos. Because neutrinos are only weakly interacting with other particles of matter, neutrino detectors must be very large in order to detect a significant number of neutrinos. Neutrino detectors are often built underground to isolate the detector from cosmic rays and other background radiation.[43] The field of neutrino astronomy is still very much in its infancy – the only confirmed extraterrestrial sources so far are the Sun and supernova SN1987A. Various detection methods have been used. Super Kamiokande is a large volume of water surrounded by phototubes that watch for the Cherenkov radiation emitted when an incoming neutrino creates an electron or muon in the water. The Sudbury Neutrino Observatory is similar, but uses heavy water as the detecting medium. Other detectors have consisted of large volumes of chlorine or gallium which are periodically checked for excesses of argon or germanium, respectively, which are created by neutrinos interacting with the original substance. MINOS uses a solid plastic scintillator watched by phototubes, Borexino uses a liquid pseudocumene scintillator also watched by phototubes while the proposed NOνA detector will use liquid scintillator watched by avalanche photodiodes."[44]
Gamma rays
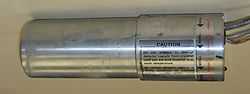
"Germanium detectors are mostly used for spectroscopy in nuclear physics. ... germanium can have a depleted, sensitive thickness of centimeters, and therefore can be used as a total absorption detector for gamma rays up to few MeV. These detectors are also called high-purity germanium detectors (HPGe) or hyperpure germanium detectors. ... germanium crystals were doped with lithium ions (Ge(Li)), in order to produce an intrinsic region in which the electrons and holes would be able to reach the contacts and produce a signal. ... HPGe detectors commonly use lithium diffusion to make an n+ ohmic contact, and boron implantation to make a p+ contact. Coaxial detectors with a central n+ contact are referred to as n-type detectors, while p-type detectors have a p+ central contact. The thickness of these contacts represents a dead layer around the surface of the crystal within which energy depositions do not result in detector signals."[45]
X-rays
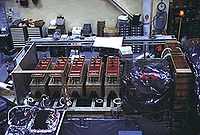
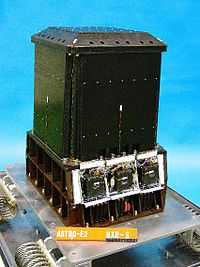
Detectors such as the X-ray detector at right collect individual X-rays (photons of X-ray light), count them, discern the energy or wavelength, or how fast they are detected.
"X-ray detectors are devices used to measure the flux, spatial distribution, spectrum or other properties of X-rays. They vary in shape and function depending on their purpose. Some common principles used to detect X-rays include the ionization of gas, the conversion to visible light in a scintillator and the production of electron-hole pairs in a semiconductor detector."[46]
"X-ray spectra can be measured either by energy dispersive or wavelength dispersive spectrometers."[46]
"X-ray detectors can be either photon counting or integrating. Photon-counting detectors measure each individual x-ray photon separately, while integrating detectors measure the total amount of energy deposited in the active region of the detector. Photon-counting detectors are normally more sensitive since they do not suffer from thermal and readout noise in the same way. An other advantage is that they can be set to count only photons in a certain energy range, or even messure the energy of each absorbed photon. Integrating detectors are normally simpler and can handle much higher photon fluxes."[46]
"Aluminum nitride has the widest band-gap of any compound semiconductor and offers the potential of making ‘‘solar-blind’’ X-ray detectors, i.e., detectors insensitive to the solar visible and ultraviolet (UV) radiation."[39]
Ultraviolets
"The dispersed ultraviolet light [from the FUSE telescope] is detected by two microchannel plate intensified double delay-line detectors, whose surfaces are curved to match the curvature of the focal plane.[47]"[48]
"Two mirror segments are coated with silicon carbide for reflectivity at the shortest ultraviolet wavelengths, and two mirror segments are coated with lithium fluoride over aluminum that reflects better at longer wavelengths." Each segment such as with silicon carbide has a dedicated microchannel plate. The other microchannel plates are for the lithium fluoride mirror system.
"LYRA will monitor the solar irradiance in four UV passbands. They have been chosen for their relevance to solar physics, aeronomy and Space Weather:"[49]
- the 115-125 nm Lyman-α channel,
- the 200-220 nm Herzberg continuum channel,
- the Aluminium filter channel (17-50 nm) including He II at 30.4 nm, and
- the Zirconium filter channel (1-20 nm).
"Diamond sensors make the instruments radiation-hard and solar-blind: their high bandgap energy makes them quasi-insensitive to visible light".[49]
Solar blind Cs-Te and Cs-I photocathode materials are sensitive to vacuum-UV and ultraviolet [and] [i]nsensitive to visible light and infrared (CsTe has cutoff at 320 nm, CsI at 200 nm)."[50]
"Magnesium fluoride transmits ultraviolet down to 115 nm. [But, it is] [h]ygroscopic, though less than other alkali halides usable for UV windows."[50]
Opticals
"Transition radiation (TR) is a form of electromagnetic radiation emitted when a charged particle passes through inhomogeneous media, such as a boundary between two different media. This is in contrast to Cherenkov radiation, which occurs when a charged particle passes through a homogeneous dielectric medium at a speed greater than the phase velocity of electromagnetic waves in that medium."[51]
"Optical Transition radiation is produced by relativistic charged particles when they cross the interface of two media of different dielectric constants. The emitted radiation is the homogeneous difference between the two inhomogeneous solutions of Maxwell's equations of the electric and magnetic fields of the moving particle in each medium separately. In other words, since the electric field of the particle is different in each medium, the particle has to "shake off" the difference when it crosses the boundary. The total energy loss of a charged particle on the transition depends on its Lorentz factor and is mostly directed forward, peaking at an angle of the order of
relative to the particle's path. The intensity of the emitted radiation is roughly proportional to the particle's energy
."[51]
"A transition radiation detector (TRD) is a particle detector using the -dependent threshold of transition radiation in a stratified material. It contains many layers of materials with different indices of refraction. At each interface between materials, the probability of transition radiation increases with the relativistic gamma factor. Thus particles with large
give off many photons, and small
give off few. For a given energy, this allows a discrimination between a lighter particle (which has a high
and therefore radiates) and a heavier particle (which has a low
and radiates much less)."[52]
"Multialkali (Na-K-Sb-Cs) [photocathode materials have a] wide spectral response from ultraviolet to near-infrared [where] special cathode processing can extend range to 930 nm. [These are] [u]sed in broadband spectrophotometers."[50]
"Borosilicate glass [window material] is commonly used for near-infrared to about 300 nm."[50]
Visuals
"[T]he wide-gap II-VI semiconductor ZnO doped with Co2+ (Zn1-xCoxO) ... responds to visible light ... Excitation into the intense 4T1(P) d-d band at ∼2.0 eV (620 nm) leads to Co2+/3+ ionization [with an] experimental maximum in the external photon-to-current conversion efficiencies at values well below the solid solubility of Co2+ in ZnO."[53]
Violets
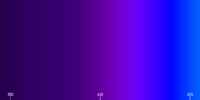
Most spacecraft designed for optical astronomy or visual astronomy carry aboard a violet or blue filter covering the wavelength range from 350-430 nm. The Solid State Imaging camera of the Galileo spacecraft uses a broad-band filter centered at 404 nm for violet astronomy.
The Hubble Space Telescope has throughout its long life used a variety of violet broad and narrow band filters for violet astronomy. The Wide Field Planetary Camera (PC-1) in use from about 1990 through 1993 carried the violet band filters: F330W, F336W, F344N, F368M, F375N, F413M, F435W, F437N, F439W, and F469N. The Wide Field Planetary Camera (PC-2) replaced PC-1 and carried the following violet filters on the same filter wheels: F300W, F336W, F343N, F375N, F380W, F390N, F410M, F437N, F439W, F450W, F467M and F469N.
The violet filter on each of the Viking Orbiters is centered at 440 nm with a range of 350-470 nm.
At right is an image of the spectral range of the Violet filter (50 to 400 nm)[54] on the Imaging Science System aboard the Voyager 1 and Voyager 2 Spacecraft, as defined by the instrument descriptions of the Narrow Angle Camera and Wide Angle Camera.
Blues
In about 1981 "an efficient blue- and violet-sensitive RCA CCD did appear on the market."[55]
"To allow for the maximum number of high-efficiency coatings it was decided to separate the light into two separate spectrographs using a dichroic filter located immediately behind the entrance aperture of slit. The dichroic, mounter at 45°, reflects blue and violet light and transmits red and near-infrared light."[55]
The blue- and violet-sensitive CCD successfully detected the helium lines from 501.5 to 318.8 nm.[55]
"The MIC (Microchannel plate Intensified CCD (Charge Coupled Device)) detector ... [has a] measured resolution of the detector system [of] 18 micrometers FWHM at 490 nm. [It is] for the ESA X-Ray Multi Mirror Mission (XMM), where the MIC has been accepted as the blue detector for the incorporated Optical Monitor (OM)."[56]
"A0620-00 [is observed] with the [Faint Object Spectrograph] FOS blue detector" while aboard the Hubble Space Telescope.[57]
Cyans
The Wide Field/Planetary Camera (PC-1) had an F469N, F487N, and F492M cyan filters in the filter wheel.[58]
The Wide Field Planetary Camera (PC-2) replaced PC-1 on the Hubble Space Telescope and carried the following cyan filters on the same filter wheels: F467M, F469N, F487N.[58]
The Advanced Camera for Surveys carried an F475W broadband cyan filter.[58]
The Faint Object Camera (FOC) carries F470M, F480LP, and F486N cyan filters.[58]
Greens
The Wide Field Planetary Camera (PC-1) of the Hubble Space Telescope was in use from about 1990 through 1993. It carried 48 filters on 12 filter wheels of four each. For the green band, these were the F492M, F502N, F517N, F547M, and the F555W. Those ending in 'N' are narrow-band filters.[58]
One of these filters is F492M which allows imaging with the [O III]λλ4959,5007 and its adjacent green continuum. The filter band pass is centered at 490.6 nm with a full-width at half maximum (FWHM) of 36.4 nm.[59]
"The F492M filter also includes Hβ.[59]
The F502N is centered at 501.85 nm with a band pass of 2.97 nm. The F547M is centered at 546.1 nm with a band pass of 43.8 nm.
The Wide Field Planetary Camera (PC-2) replaced PC-1 and carried the following filters on the same filter wheels: F467M, F502N, F547M, F555W, and the F569W.[58] In December 1993 PC-1 was replaced with PC-2 and the HST was declared operational on January 13, 1994.[60]
Onboard the HST is the Faint Object Camera (FOC) which carries filters for green astronomy: F470M, F480LP, F501N, F502N, and the F550M.[58]
"The Wide Field Camera 3 (WFC3) is the Hubble Space Telescope's last and most technologically advanced instrument to take images in the visible spectrum. It was installed as a replacement for the Wide Field and Planetary Camera 2 during the first spacewalk of Space Shuttle mission STS-125 on May 14, 2009."[61]
Yellows
"[T]he #8 yellow filter is used to show Mars's maria and Jupiter's belts.[62]"[63]
Initially the Hubble Space Telescope had the Wide Field/Planetary Camera (WF/PC-1) aboard where the F555W, F569W, F588N, and F606W cover the entire yellow portion of the electromagnetic spectrum.
The Hubble's Faint Object Camera (FOC) uses F550M and F600M which cover from either side.
The Wide Field and Planetary Camera (WFPC2) replaced PC-1 and used F555W, F569W, F588N and F606W filters.
Oranges
The WF/PC-1 filters available for orange astronomy are the F588N, F606W, and F622W. The FOC uses the F600M and F630M. The WFPC2 uses the F588N, F606W, and F622W.
Reds
The following WF/PC-1 filters are available for red astronomy: F606W, F622W, F631N, F648M, F656N, F658N, F664N, F673N, F675W, F702W, F718M, and F725LP.
The FOC uses the F630M for the shorter wavelength red rays.
The Hubble WFPC2 uses F606W, F622W, F631N, F656N, F658N, F673N, F675W, F702W, and F775W.
Infrareds
"An infrared detector is [usually one of] two main types ... thermal and photonic (photodetectors). The thermal effects of the incident IR radiation can be followed through many temperature dependent phenomena. Bolometers and microbolometers are based on changes in resistance. Thermocouples and thermopiles use the thermoelectric effect. Golay cells follow thermal expansion. In IR spectrometers the pyroelectric detectors are the most widespread."[64]
"The response time and sensitivity of photonic detectors can be much higher, but usually these have to be cooled to cut thermal noise. The materials in these are semiconductors with narrow band gaps. Incident IR photons can cause electronic excitations. In photoconductive detectors, the resistivity of the detector element is monitored. Photovoltaic detectors contain a p-n junction on which photoelectric current appears upon illumination."[64]
The Hubble PC-1 used the F785LP, F791W, F814W, F850LP, F875M, F889N, F1042M, and F1083N filters for infrared astronomy. The PC-2 used F785LP, F791W, F814W, F850LP, F953N, and F1042M.
Submillimeters
"Metal-mesh filters have many applications for use in the far infrared (FIR)[65] and submillimeter regions of the electromagnetic spectrum. These filters have been used in FIR and submillimeter astronomical instruments for over 4 decades,[66] in which they serve two main purposes: bandpass or low-pass filters are cooled and used to lower the noise equivalent power of cryogenic bolometers (detectors) by blocking excess thermal radiation outside of the frequency band of observation,[67] and bandpass filters can be used to define the observation band of the detectors. Metal-mesh filters can also be designed for use at 45° to split an incoming optical signal into several observation paths, or for use as a polarizing half wave plate.[68]"[69]
Radios
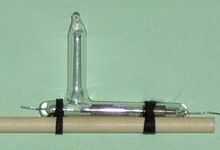
"The coherer ... consists of a tube or capsule containing two electrodes spaced a small distance apart, with metal filings in the space between them. When a radio frequency signal is applied to the device, the initial high resistance of the filings reduces, allowing an electric current to flow through it."[70]
Superluminals
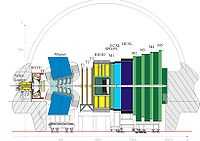
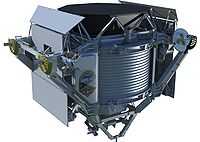
"Most Cherenkov detectors aim at recording the Cherenkov light produced by a primary charged particle. Some sensor technologies explicitly aim at Cherenkov light produced (also) by secondary particles, be it incoherent emission as occurring in an electromagnetic particle shower or by coherent emission, example Askaryan effect."[71]
"Cherenkov radiation is not only present in the range of visible light or UV light but also in any frequency range where the emission condition can be met i.e. in the radiofrequency range."[71]
"Different levels of information can be used. A binary information can be based on the absence or presence of detected Cherenkov radiation. The amount or the direction of Cherenkov light can be used. In contrast to a scintillation counter the light production is instantaneous."[71]
"Cherenkov threshold detectors have been used for fast timing and Time of flight measurements in particle physics experiments. More elaborate designs use the amount of light produced. Recording light from both primary and secondary particles, for a Cherenkov calorimeter the total light yield is proportional to the incident particle energy."[71]
"Using the light direction are differential Cherenkov detectors. Recording individual Cherenkov photon locations on a position-sensitive sensor area, RICH detectors then reconstruct Cherenkov angles from the recorded patterns. As RICH detectors hence provide information on the particle velocity, if the momentum of the particle is also known (from magnetic bending), combining these two informations enables the particle mass to be deduced so that the particle type can be identified."[71]
"A Ring-imaging Cherenkov (RICH) detector is a device that allows the identification of electrically charged subatomic particle types through the detection of the Cherenkov radiation emitted (as photons) by the particle in traversing a medium with refractive index > 1. The identification is achieved by measurement of the angle of emission,
, of the Cherenkov radiation, which is related to the charged particle's velocity
by
where is the speed of light."[72]
"The LHCb experiment on the Large Hadron Collider uses two RICH detectors for differentiating between pions and kaons.[73] The first (RICH-1) is located immediately after the Vertex Locator (VELO) around the interaction point and is optimised for low-momentum particles and the second (RICH-2) is located after the magnet and particle-tracker layers and optimised for higher-momentum particles.[74]"[72]
"The Alpha Magnetic Spectrometer device AMS-02, recently mounted on the International Space Station uses a RICH detector in combination with other devices to analyze cosmic rays."[72]
Plasma objects
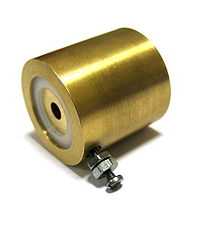
"A Faraday cup is a metal (conductive) cup designed to catch charged particles in vacuum. The resulting current can be measured and used to determine the number of ions or electrons hitting the cup.[75]"
"When a beam or packet of ions hits the metal it gains a small net charge while the ions are neutralized. The metal can then be discharged to measure a small current equivalent to the number of impinging ions. Essentially the faraday cup is part of a circuit where ions are the charge carriers in vacuum and the faraday cup is the interface to the solid metal where electrons act as the charge carriers (as in most circuits). By measuring the electrical current (the number of electrons flowing through the circuit per second) in the metal part of the circuit the number of charges being carried by the ions in the vacuum part of the circuit can be determined. For a continuous beam of ions (each with a single charge)
where is the number of ions observed in a time
(in seconds),
is the measured current (in amperes) and
is the elementary charge (about 1.60 × 10−19 C). Thus, a measured current of one nanoamp (10−9 A) corresponds to about 6 billion ions striking the faraday cup each second."[76]
Gaseous objects
"A gas detector is a device which detects the presence of various gases within an area"[77] or volume.
"The combination of nanotechnology and microelectromechanical systems (MEMS) technology allows the production of a hydrogen microsensor that functions properly at room temperature. One type of MEMS-based hydrogen sensor is coated with a film consisting of nanostructured indium oxide (In2O3) and tin oxide (SnO2).[78] A typical configuration for mechanical Pd-based hydrogen sensors is the usage of a free-standing cantilever that is coated with Pd.[79][80] In the presence of H2, the Pd layer expands and thereby induces a stress that causes the cantilever to bend. Pd-coated nano-mechanical resonators have also been reported in literature, relying on the stress-induced mechanical resonance frequency shift caused by the presence of H2 gas. In this case, the response speed was enhanced through the use of a very thin layer of Pd (20 nm). Moderate heating was presented as a solution to the response impairment observed in humid conditions.[81]"[82]
Liquid objects
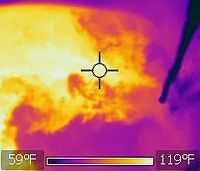
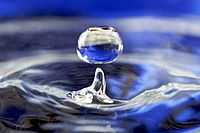
"A liquid is made up of tiny vibrating particles of matter, such as atoms and molecules, held together by intramolecular bonds. ... Although liquid water is abundant on Earth, this state of matter is actually the least common in the known universe, because liquids require a relatively narrow temperature/pressure range to exist."[83]
The first image at right shows liquid water using an infrared detector, but information confirming the presence of liquid water solely from the infrared image is inferred.
The image at left uses a visual radiation detector to record a meteor collision with liquid water.
"Reconstructions of seismic waves in the deep interior of the Earth show that there are no S-waves in the outer core. This indicates that the outer core is liquid, because liquids cannot support shear. The outer core is liquid, and the motion of this highly conductive fluid generates the Earth's field (see geodynamo)."[84]
Rocky objects
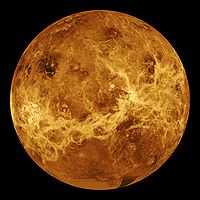
Often a rocky object is detected by the observation of impact craters usually using visual astronomy as part of crater astronomy.
"A number of bright spots with a bluish tinge are visible ... These are relatively recent impact craters. Some of the bright craters have bright streaks ... emanating from them. Bright features such as these are caused by the presence of freshly crushed rock material that was excavated and deposited during the highly energetic collision of a meteoroid with Mercury to form an impact crater."[85]
The object at left is detected to be a rocky object using radar astronomy.
"The advantages of radar in planetary astronomy result from (1) the observer's control of all the attributes of the coherent signal used to illuminate the target, especially the wave form's time/frequency modulation and polarization; (2) the ability of radar to resolve objects spatially via measurements of the distribution of echo power in time delay and Doppler frequency; (3) the pronounced degree to which delay-Doppler measurements constrain orbits and spin vectors; and (4) centimeter-to-meter wavelengths, which easily penetrate optically opaque planetary clouds and cometary comae, permit investigation of near-surface macrostructure and bulk density, and are sensitive to high concentrations of metal or, in certain situations, ice."[86]
Astrochemistry
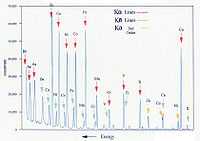
"Each element has electronic orbitals of characteristic energy. Following removal of an inner electron by an energetic photon provided by a primary radiation source, an electron from an outer shell drops into its place. There are a limited number of ways in which this can happen ... The main transitions are given names: an L→K transition is traditionally called Kα, an M→K transition is called Kβ, an M→L transition is called Lα, and so on. Each of these transitions yields a fluorescent photon with a characteristic energy equal to the difference in energy of the initial and final orbital."[87]
Compounds
"[T]he detection of absorption by interstellar hydrogen fluoride (HF) [in the submillimeter band occurs] along the sight line to the submillimeter continuum sources W49N and W51."[88]
"[A]bsorption features in the submillimeter spectrum of Mars ... are due to the H2O (110-101) and 13CO (5-4) rotational transitions."[89]
Alloys
"A metal detector is a device which responds to metal that may not be readily apparent. The simplest form of a metal detector consists of an oscillator producing an alternating current that passes through a coil producing an alternating magnetic field. If a piece of electrically conductive metal is close to the coil, eddy currents will be induced in the metal, and this produces a magnetic field of its own. If another coil is used to measure the magnetic field (acting as a magnetometer), the change in the magnetic field due to the metallic object can be detected."[90]
"Modern top models are fully computerized, using integrated circuit technology to allow the user to set sensitivity, discrimination, track speed, threshold volume, notch filters, etc., and hold these parameters in memory for future use. Compared to just a decade ago, detectors are lighter, deeper-seeking, use less battery power, and discriminate better."[90]
"Coil designers also tried out innovative designs. The original induction balance coil system consisted of two identical coils placed on top of one another. Compass Electronics produced a new design: two coils in a D shape, mounted back-to-back to form a circle. This system was widely used in the 1970s, and both concentric and D type (or widescan as they became known) had their fans. Another development was the invention of detectors which could cancel out the effect of mineralization in the ground. This gave greater depth, but was a non-discriminate mode. It worked best at lower frequencies than those used before, and frequencies of 3 to 20 kHz were found to produce the best results. Many detectors in the 1970s had a switch which enabled the user to switch between the discriminate mode and the non-discriminate mode. Later developments switched electronically between both modes. The development of the induction balance detector would ultimately result in the motion detector, which constantly checked and balanced the background mineralization."[90]
"At the same time, developers were looking at using a different technique in metal detection called pulse induction. Unlike the beat frequency oscillator or the induction balance machines which both used a uniform alternating current at a low frequency, the pulse induction machine simply fired a high-voltage pulse of signal into the ground. In the absence of metal, the pulse decayed at a uniform rate, and the time it took to fall to zero volts could be accurately measured. However, if metal was present when the machine fired, a small current would flow in the metal, and the time for the voltage to drop to zero would be increased. These time differences were minute, but the improvement in electronics made it possible to measure them accurately and identify the presence of metal at a reasonable distance. These new machines had one major advantage: they were completely impervious to the effects of mineralization, and rings and other jewelry could now be located even under highly-mineralized black sand."[90]
Sun (star)
"A sun sensor is a device that senses the direction to the Sun. This can be as simple as some solar cells and shades, or as complex as a steerable telescope, depending on mission requirements."[8]
Earth
"An earth sensor is a device that senses the direction to the Earth. It is usually an infrared camera; now the main method to detect attitude is the star tracker, but earth sensors are still integrated in satellites for their low cost and reliability."[8]
Stars
"A star tracker is an optical device that measures the position(s) of star(s) using photocell(s) or a camera.[91]"[8]
"Star trackers, which require high sensitivity, may become confused by sunlight reflected from the spacecraft, or by exhaust gas plumes from the spacecraft thrusters (either sunlight reflection or contamination of the star tracker window). Star trackers are also susceptible to a variety of errors (low spatial frequency, high spatial frequency, temporal, ...) in addition to a variety of optical sources of error (spherical aberration, chromatic aberration, ...). There are also many potential sources of confusion for the star identification algorithm (planets, comets, supernovae, the bimodal character of the point spread function for adjacent stars, other nearby satellites, point-source light pollution from large cities on Earth, ...). There are roughly 57 bright navigational stars in common use. However, for more complex missions, entire star field databases are used to determine spacecraft orientation. A typical star catalog for high-fidelity attitude determination is originated from a standard base catalog (for example from the United States Naval Observatory) and then filtered to remove problematic stars, for example due to apparent magnitude variability, color index uncertainty, or a location within the Hertzsprung-Russell diagram implying unreliability. These types of star catalogs can have thousands of stars stored in memory on board the spacecraft, or else processed using tools at the ground station and then uploaded."[8]
Geography
Occasionally, a detector needs a specific geographic property for optimal function.
Large surface area
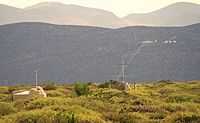
“The Pierre Auger Observatory is an international cosmic ray observatory designed to detect ultra-high-energy cosmic rays: single sub-atomic particles (protons or atomic nuclei) with energies beyond 1020 eV (about the energy of a tennis ball traveling at 80 km/h). These high energy particles have an estimated arrival rate of just 1 per km2 per century, therefore the Auger Observatory has created a detection area the size of Rhode Island — over 3,000 km2 (1,200 sq mi) — in order to record a large number of these events. It is located in western Argentina's Mendoza Province, in one of the South American Pampas.”[33]
Next to water
.jpg)
"The Big Bear Solar Observatory (BBSO) is a solar observatory located on the north side of Big Bear Lake in the San Bernardino Mountains of southwestern San Bernardino County, California (USA), approximately 120 kilometers (75 mi) east of downtown Los Angeles."[92]
"The location at Big Bear Lake is optimal due to the clarity of the sky and the presence a body of water. The lake surface is about 2,055 meters (6,742 ft) above sea level, putting it above a significant portion of the atmosphere. The main observatory building is in the open waters of the lake, and was originally reached by boat, though a causeway was added later.[93] The water provides a cooling effect on the atmosphere surrounding the building and eliminates ground heat radiation waves that normally would cause optical aberrations."[92]
Underground
"Neutrino detectors [such as the Sudbury Neutrino Observatory shown in neutrino detectors] are often built underground to isolate the detector from cosmic rays and other background radiation.[43]"[44]
Under ice
"IceCube [under the ice at the Amundsen-Scott South Pole Station in Antarctica ] contains thousands of spherical optical sensors called Digital Optical Modules (DOMs), each with a photomultiplier tube (PMT)[94] and a single board data acquisition computer which sends digital data to the counting house on the surface above the array.[95]"[96]
Under water
"ANTARES is the name of a neutrino detector residing 2.5 km under the Mediterranean Sea off the coast of Toulon, France. It is designed to be used as a directional Neutrino Telescope to locate and observe neutrino flux from cosmic origins in the direction of the Southern Hemisphere of the Earth, a complement to the southern hemisphere neutrino detector IceCube that detects neutrinos from the North."[97]
History
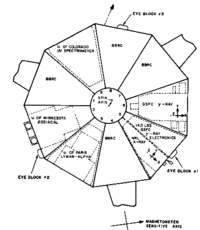
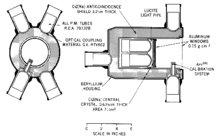
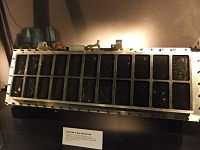
"The wheel of the [OSO 5] satellite carried, amongst other experiments, a CsI crystal scintillator. The central crystal was 0.635 cm thick, had a sensitive area of 70 sq-cm, and was viewed from behind by a pair of photomultiplier tubes. The shield crystal had a wall thickness of 4.4 cm and was viewed by 4 photomultipliers. The field of view was ~ 40 degrees. The energy range covered was 14-254 keV. There were 9 energy channels: the first covering 14-28 keV and the others equally spaced from 28-254 keV. In-flight calibration was done with an 241 Am source. The instrument was designed primarily for observation of solar X-ray bursts. A secondary interest was the measurement of the intensity, spectrum, and spatial distribution of the diffuse cosmic background. The data produced a spectrum of the diffuse background over the energy range 14-200 keV."[98]
Mathematics
"The energy differences between levels in the Bohr model, and hence the wavelengths of emitted/absorbed photons, is given by the Rydberg formula[99]:
where n is the initial energy level, n′ is the final energy level, and R is the Rydberg constant. Meaningful values are returned only when n is greater than n′ and the limit of one over infinity is taken to be zero."[100]
Physics
"A relativistic jet coming out of the center of an active galactic nucleus is moving along AB with a velocity v. We are observing the jet from the point O. At time a light ray leaves the jet from point A and another ray leaves at time
from point B. Observer at O receives the rays at time
and
respectively."[101]
-
, where
Apparent transverse velocity along CB,
-
, where
"If (i.e. when velocity of jet is close to the velocity of light) then
despite the fact that
. And of course
means apparent transverse velocity along CB, the only velocity on sky that we can measure, is larger than the velocity of light in vacuum, i.e. the motion is apparently superluminal."[101]
Gas-chamber detectors
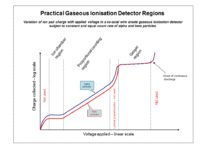
"X-rays going through a gas will ionize it, producing positive ions and free electrons. An incoming photon will create a number of such ion pairs proportional to its energy. If there is an electric field in the gas chamber ions and electrons will move in different directions and thereby cause a detectable current. The behaviour of the gas will depend on the applied voltage and the geometry of the chamber."[46]
"Ionization chambers use a relatively low electric field of about 100 V/cm to extract all ions and electrons before they recombine.[102] This gives a steady current proportional to the dose rate the gas is exposed to."[46]
"Proportional counters use a geometry with a thin positively charged anode wire in the center of a cylindrical chamber. Most of the gas volume will act as an ionization chamber, but in the region closest to the wire the electric field is high enough to make the electrons ionize gas molecules. This will create an avalanche effect greatly increasing the output signal. Since every electron cause an avalanche of approximately the same size the collected charge is proportional to the number of ion pairs created by the absorbed x-ray. This makes it possible to measure the energy of each incoming photon."[46]
"Gas detectors are usually single pixel detectors measuring only the average dose rate over the gas volume or the number of interacting photons ..., but they can be made spatially resolving by having many crossed wires in a wire chamber."[46]
Lithium-drifted silicon detectors
"Since the 1970s, new semiconductor detectors have been developed (silicon or germanium doped with lithium: Si(Li) or Ge(Li)). X-ray photons are converted to electron-hole pairs in the semiconductor and are collected to detect the X-rays. When the temperature is low enough (the detector is cooled by Peltier effect or even cooler liquid nitrogen), it is possible to directly determine the X-ray energy spectrum; this method is called energy dispersive X-ray spectroscopy (EDX or EDS); it is often used in small X-ray fluorescence spectrometers. These detectors are sometimes called "solid state detectors". Detectors based on cadmium telluride (CdTe) and its alloy with zinc, cadmium zinc telluride, have an increased sensitivity, which allows lower doses of X-rays to be used."[46]
Scintillation detectors
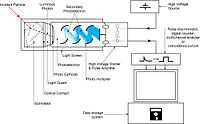
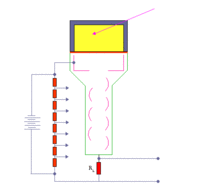
"Some materials such as sodium iodide (NaI) can "convert" an X-ray photon to a visible photon; an electronic detector can be built by adding a photomultiplier. These detectors are called "scintillators", filmscreens or "scintillation counters"."[46]
"A scintillator is a material, which exhibits scintillation—the property of luminescence[103] when excited by ionizing radiation. Luminescent materials, when struck by an incoming particle, absorb its energy and scintillate, i.e., reemit the absorbed energy in the form of light."[104] Here, "particle" refers to "ionizing radiation" and can refer either to charged particulate radiation, such as electrons and heavy charged particles, or to uncharged radiation, such as photons and neutrons, provided that they have enough energy to induce ionization.
"A scintillation detector or scintillation counter is obtained when a scintillator is coupled to an electronic light sensor such as a photomultiplier tube (PMT) or a photodiode. PMTs absorb the light emitted by the scintillator and reemit it in the form of electrons via the photoelectric effect. The subsequent multiplication of those electrons (sometimes called photo-electrons) results in an electrical pulse which can then be analyzed and yield meaningful information about the particle that originally struck the scintillator."[104]
"When a charged particle strikes the scintillator, its atoms are excited and photons are emitted. These are directed at the photomultiplier tube's photocathode, which emits electrons by the photoelectric effect. These electrons are electrostatically accelerated and focused by an electrical potential so that they strike the first dynode of the tube. The impact of a single electron on the dynode releases a number of secondary electrons which are in turn accelerated to strike the second dynode. Each subsequent dynode impact releases further electrons, and so there is a current amplifying effect at each dynode stage. Each stage is at a higher potential than the previous to provide the accelerating field. The resultant output signal at the anode is in the form of a measurable pulse for each photon detected at the photocathode, and is passed to the processing electronics. The pulse carries information about the energy of the original incident radiation on the scintillator. Thus both intensity and energy of the radiation can be measured."[105]
"The time evolution of the number of emitted scintillation photons N in a single scintillation event can often be described by the linear superposition of one or two exponential decays. For two decays, we have the form:[103]
where τf and τs are the fast (or prompt) and the slow (or delayed) decay constants. Many scintillators are characterized by 2 time components: one fast (or prompt), the other slow (or delayed). While the fast component usually dominates, the relative amplitude A and B of the two components depend on the scintillating material. Both of these components can also be a function the energy loss dE/dx. In cases where this energy loss dependence is strong, the overall decay time constant varies with the type of incident particle. Such scintillators enable pulse shape discrimination, i.e., particle identification based on the decay characteristics of the PMT electric pulse. For instance, when BaF2 is used, γ rays typically excite the fast component, while α particles excite the slow component: it is thus possible to identify them based on the decay time of the PMT signal."[104]
“Scintillation neutron detectors include liquid organic scintillators,[106] crystals,[107][108] plastics, glass[109] and scintillation fibers.[110]”[36]
Semiconductor detectors
"A semiconductor detector is a device that uses a semiconductor (usually silicon or germanium) to detect traversing charged particles or the absorption of photons. In the field of particle physics, these detectors are usually known as silicon detectors. When their sensitive structures are based on a single diode, they are called semiconductor diode detectors. When they contain many diodes with different functions, the more general term semiconductor detector is used. Semiconductor detectors have found broad application during recent decades, in particular for gamma and X-ray spectrometry and as particle detectors."[45]
"[R]adiation is measured by means of the number of charge carriers set free in the detector, which is arranged between two electrodes. Ionizing radiation produces free electrons and holes. The number of electron-hole pairs is proportional to the energy transmitted by the radiation to the semiconductor. As a result, a number of electrons are transferred from the valence band to the conduction band, and an equal number of holes are created in the valence band. Under the influence of an electric field, electrons and holes travel to the electrodes, where they result in a pulse that can be measured in an outer circuit, as described by the Shockley-Ramo Theorem. The holes travel in the opposite direction and can also be measured. As the amount of energy required to create an electron-hole pair is known, and is independent of the energy of the incident radiation, measuring the number of electron-hole pairs allows the energy of the incident radiation to be found.[111]"[45]
“Semiconductors have been used for neutron detection.[112]”[36]
Silicon drift detectors
"Silicon drift detectors (SDDs), produced by conventional semiconductor fabrication, now provide a cost-effective and high resolving power radiation measurement. Unlike conventional X-ray detectors, such as Si(Li)s, they do not need to be cooled with liquid nitrogen."[46]
Scintillator plus semiconductor detectors
"With the advent of large semiconductor array detectors it has become possible to design detector systems using a scintillator screen to convert from X-rays to visible light which is then converted to electrical signals in an array detector. ... The array consists of a sheet of glass covered with a thin layer of silicon that is in an amorphous or disordered state. At a microscopic scale, the silicon has been imprinted with millions of transistors arranged in a highly ordered array, like the grid on a sheet of graph paper. Each of these thin film transistors (TFTs) is attached to a light-absorbing photodiode making up an individual pixel (picture element). Photons striking the photodiode are converted into two carriers of electrical charge, called electron-hole pairs. Since the number of charge carriers produced will vary with the intensity of incoming light photons, an electrical pattern is created that can be swiftly converted to a voltage and then a digital signal, which is interpreted by a computer to produce a digital image. Although silicon has outstanding electronic properties, it is not a particularly good absorber of X-ray photons. For this reason, X-rays first impinge upon scintillators made from such materials as gadolinium oxysulfide or caesium iodide. The scintillator absorbs the X-rays and converts them into visible light photons that then pass onto the photodiode array."[46]
Research
Hypothesis:
- Radiation detectors can be built to differentiate between superluminal, luminal, and subluminal radiations.
Control groups
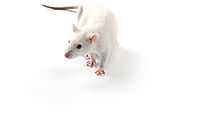
The findings demonstrate a statistically systematic change from the status quo or the control group.
“In the design of experiments, treatments [or special properties or characteristics] are applied to [or observed in] experimental units in the treatment group(s).[113] In comparative experiments, members of the complementary group, the control group, receive either no treatment or a standard treatment.[114]"[115]
Proof of concept
Def. a “short and/or incomplete realization of a certain method or idea to demonstrate its feasibility"[116] is called a proof of concept.
Def. evidence that demonstrates that a concept is possible is called proof of concept.
The proof-of-concept structure consists of
- background,
- procedures,
- findings, and
- interpretation.[117]
Proof of technology
"[T]he objective of a proof of technology is to determine the solution to some technical problem, such as how two systems might be integrated or that a certain throughput can be achieved with a given configuration."[118]
Def.
- "[a]n original object or form which is a basis for other objects, forms, or for its models and generalizations",[119]
- "[a]n early sample or model built to test a concept or process",[119] or
- "[a]n instance of a category or a concept that combines its most representative attributes"[119] is called a prototype.
Def. "[t]o test something using the conditions that it was designed to operate under, especially out in the real world instead of in a laboratory or workshop"[120] is called "field-test", or a field test.
A "proof-of-technology prototype ... typically implements one critical scenario to exercise or stress the highest-priority requirements."[121]
"[A] proof-of-technology test demonstrates the system can be used"[122].
"The strongest proof of technology performance is based on consistency among multiple lines of evidence, all pointing to similar levels of risk reduction."[123]
See also
References
- 1 2 "Radiation damage, In: Wikipedia". San Francisco, California: Wikimedia Foundation, Inc. March 16, 2013. Retrieved 2013-05-24.
- ↑ "Radiation hardening, In: Wikipedia". San Francisco, California: Wikimedia Foundation, Inc. May 8, 2013. Retrieved 2013-05-24.
- ↑ "Detector (radio), In: Wikipedia". San Francisco, California: Wikimedia Foundation, Inc. May 15, 2013. Retrieved 2013-05-25.
- ↑ "detector, In: Wiktionary". San Francisco, California: Wikimedia Foundation, Inc. May 30, 2012. Retrieved 2012-06-19.
- ↑ "Particle detector, In: Wikipedia". San Francisco, California: Wikimedia Foundation, Inc. April 22. 2012. Retrieved 2012-06-19.
- ↑ "sensor, In: Wiktionary". San Francisco, California: Wikimedia Foundation, Inc. June 12, 2012. Retrieved 2012-06-19.
- ↑ "Sense, In: Wikipedia". San Francisco, California: Wikimedia Foundation, Inc. May 4, 2013. Retrieved 2013-05-17.
- 1 2 3 4 5 6 7 8 9 "Attitude control, In: Wikipedia". San Francisco, California: Wikimedia Foundation, Inc. May 17, 2013. Retrieved 2013-05-17.
- ↑ Hemispherical Resonator Gyros, Northrop Grumman Corp.
- ↑ "Gadolinium oxysulfide, In: Wikipedia". San Francisco, California: Wikimedia Foundation, Inc. March 16, 2013. Retrieved 2013-05-20.
- ↑ P. Capper (1994). Properties of Narrow-Gap Cadmium-Based Compounds. London, UK: INSPEC, IEE. ISBN 0-85296-880-9.
- ↑ "Cadmium telluride, In: Wikipedia". San Francisco, California: Wikimedia Foundation, Inc. May 5, 2013. Retrieved 2013-05-20.
- 1 2 3 4 5 Henric S. Krawczynski ; Ira Jung ; Jeremy S. Perkins ; Arnold Burger ; Michael Groza (October 21, 20042004). Thick CZT Detectors for Space-Borne X-ray Astronomy, In: Hard X-Ray and Gamma-Ray Detector Physics VI, 1. 5540. Denver, Colorado USA: The International Society for Optical Engineering. pp. 13. doi:10.1117/12.558912. http://arxiv.org/pdf/astro-ph/0410077. Retrieved 2013-05-20.
- 1 2 3 4 5 6 "Stopping power (particle radiation), In: Wikipedia". San Francisco, California: Wikimedia Foundation, Inc. April 11, 2013. Retrieved 2013-04-11.
- 1 2 3 4 "Neutron detection, In: Wikipedia". San Francisco, California: Wikimedia Foundation, Inc. March 17, 2013. Retrieved 2013-05-22.
- 1 2 "Radiography, In: Wikipedia". San Francisco, California: Wikimedia Foundation, Inc. May 18, 2013. Retrieved 2013-05-22.
- ↑ "3D single-object recognition, In: Wikipedia". San Francisco, California: Wikimedia Foundation, Inc. August 22, 2012. Retrieved 2013-05-22.
- ↑ "Outline of object recognition, In: Wikipedia". San Francisco, California: Wikimedia Foundation, Inc. April 5, 2013. Retrieved 2013-05-22.
- ↑ "Xenon arc lamp, In: Wikipedia". San Francisco, California: Wikimedia Foundation, Inc. May 6, 2013. Retrieved 2013-05-22.
- ↑ "Continuous spectrum, In: Wikipedia". San Francisco, California: Wikimedia Foundation, Inc. March 31, 2013. Retrieved 2013-05-22.
- ↑ "Compton scattering, In: Wikipedia". San Francisco, California: Wikimedia Foundation, Inc. May 16, 2013. Retrieved 2013-05-22.
- ↑ B. A. Dolgoshein, V. N. Lebedenko & B. I. Rodionov, "New method of registration of ionizing-particle tracks in condensed matter", JETP Lett. 11(11): 351 (1970)
- ↑ "ZEPLIN-III, In: Wikipedia". San Francisco, California: Wikimedia Foundation, Inc. May 10, 2013. Retrieved 2013-05-22.
- ↑ "Absorption spectroscopy, In: Wikipedia". San Francisco, California: Wikimedia Foundation, Inc. March 13, 2013. Retrieved 2013-05-22.
- 1 2 "Band gap, In: Wikipedia". San Francisco, California: Wikimedia Foundation, Inc. May 11, 2013. Retrieved 2013-05-23.
- ↑ "Electronic band structure, In: Wikipedia". San Francisco, California: Wikimedia Foundation, Inc. May 20, 2013. Retrieved 2013-05-23.
- 1 2 C. D. Motchenbacher, J. A. Connelly (1993). Low-noise electronic system design. Wiley Interscience.
- ↑ L. B. Kish, C. G. Granqvist (November 2000). "Noise in nanotechnology". Microelectronics Reliability (Elsevier) 40 (11): 1833–37. doi:10.1016/S0026-2714(00)00063-9.
- 1 2 "Noise (electronics), In: Wikipedia". San Francisco, California: Wikimedia Foundation, Inc. March 12, 2013. Retrieved 2013-05-24.
- 1 2 3 "Voltage spike, In: Wikipedia". San Francisco, California: Wikimedia Foundation, Inc. May 15, 2013. Retrieved 2013-05-24.
- ↑ Transient Protection, LearnEMC Online Tutorial. http://www.learnemc.com/tutorials/Transient_Protection/t-protect.html
- ↑ Dennis Overbye (2009-07-24). "Hubble Takes Snapshot of Jupiter’s ‘Black Eye’". New York Times. Retrieved 2009-07-25.
- 1 2 3 "Pierre Auger Observatory, In: Wikipedia". San Francisco, California: Wikimedia Foundation, Inc. June 10, 2012. Retrieved 2012-06-19.
- 1 2 3 4 "Cloud chamber, In: Wikipedia". San Francisco, California: Wikimedia Foundation, Inc. April 15, 2013. Retrieved 2013-05-17.
- 1 2 Samuel Ting, Manuel Aguilar-Benitez, Silvie Rosier, Roberto Battiston, Shih-Chang Lee, Stefan Schael, and Martin Pohl (April 13, 2013). "Alpha Magnetic Spectrometer - 02 (AMS-02)". Washington, DC USA: NASA. Retrieved 2013-05-17.
- 1 2 3 4 5 "Neutron detector, In: Wikipedia". San Francisco, California: Wikimedia Foundation, Inc. June 6, 2012. Retrieved 2012-06-19.
- 1 2 3 4 Tsoulfanidis, Nicholas (1995 (2nd Edition)). Measurement and Detection of Radiation. Washington, D.C.: Taylor & Francis. pp. 467–501. ISBN 1-56032-317-5.
- ↑ "Alpha particle X-ray spectrometer, In: Wikipedia". San Francisco, California: Wikimedia Foundation, Inc. April 6. 2013. Retrieved 2013-05-17.
- 1 2 Alan Owens, A. Peacock (September 2004). "Compound semiconductor radiation detectors". Nuclear Instruments and Methods in Physical Research A 531 (1-2): 18-37. doi:10.1016/j.nima.2004.05.071. http://www.msri.org/people/staff/levy/files/ToPrint/owens-compound.pdf. Retrieved 2013-05-24.
- ↑ "Galileo (spacecraft), In: Wikipedia". San Francisco, California: Wikimedia Foundation, Inc. August 7, 2012. Retrieved 2012-08-11.
- ↑ Donald J. Williams (May 14, 2012). "Energetic Particles Detector (EPD)". Greenbelt, Maryland USA: NASA Goddard Space Flight Center. Retrieved 2012-08-11.
- ↑ Francis Halzen, Todor Stanev, Gaurang B. Yodh (April 1, 1997). "γ ray astronomy with muons". Physical Review D Particles, Fields, Gravitation, and Cosmology 55 (7): 4475-9. doi:10.1103/PhysRevD.55.4475. http://prd.aps.org/abstract/PRD/v55/i7/p4475_1. Retrieved 2013-01-18.
- 1 2 Ian Sample (23 January 2011). "The hunt for neutrinos in the Antarctic". The Guardian. Retrieved 2011-06-16.
- 1 2 "Neutrino detector, In: Wikipedia". San Francisco, California: Wikimedia Foundation, Inc. May 23, 2012. Retrieved 2012-06-19.
- 1 2 3 "Semiconductor detector, In: Wikipedia". San Francisco, California: Wikimedia Foundation, Inc. June 19, 2012. Retrieved 2012-06-19.
- 1 2 3 4 5 6 7 8 9 10 11 "X-ray detector, In: Wikipedia". San Francisco, California: Wikimedia Foundation, Inc. April 9, 2013. Retrieved 2013-05-17.
- ↑ D.J. Sahnow, et al. (1995-07-03). "The Far Ultraviolet Spectroscopic Explorer Mission". JHU.edu. Retrieved 2007-09-07.
- ↑ "Far Ultraviolet Spectroscopic Explorer, In: Wikipedia". San Francisco, California: Wikimedia Foundation, Inc. March 9, 2012. Retrieved 2012-06-26.
- 1 2 "LYRA, In: Wikipedia". San Francisco, California: Wikimedia Foundation, Inc. April 25, 2013. Retrieved 2013-05-24.
- 1 2 3 4 "Photomultiplier, In: Wikipedia". San Francisco, California: Wikimedia Foundation, Inc. May 14, 2013. Retrieved 2013-05-24.
- 1 2 "Transition radiation, In: Wikipedia". San Francisco, California: Wikimedia Foundation, Inc. February 28, 2013. Retrieved 2013-05-20.
- ↑ "Transition radiation detector, In: Wikipedia". San Francisco, California: Wikimedia Foundation, Inc. March 7, 2013. Retrieved 2013-05-20.
- ↑ Claire A. Johnson, Alicia Cohn, Tiffany Kaspar, Scott A. Chambers, G. Mackay Salley, and Daniel R. Gamelin (September 6, 2011). "Visible-light photoconductivity of Zn1-xCoxO and its dependence on Co2+ concentration". Physical Review B 84 (12): 8. doi:10.1103/PhysRevB.84.125203. http://prb.aps.org/abstract/PRB/v84/i12/e125203. Retrieved 2013-05-24.
- ↑ M. Benesh and F. Jepsen (August 6, 1984). "SP-474 Voyager 1 and 2 Atlas of Six Saturnian Satellites Appendix A The Voyager Mission". Washington, DC USA: NASA. Retrieved 2013-04-01.
- 1 2 3 J. B. Oke and J. E. Gunn (June 1982). "An Efficient Low Resolution and Moderate Resolution Spectrograph for the Hale Telescope". Publications of the Astronomical Society of the Pacific 94 (06): 586-94. doi:10.1086/131027.
- ↑ J. L. A. Fordham, D. A. Bone, M. K. Oldfield, J. G. Bellis, and T. J. Norton (December 1992). The MIC photon counting detector, In: Proceedings of an ESA Symposium on Photon Detectors for Space Instrumentation. European Space Agency. pp. 103-6. Bibcode: 1992ESASP.356..103F.
- ↑ Jeffrey McClintock (December 1997). Black Hole A0620-00 and Advection-Dominated Accretion, In: HST Proposal ID #7393. Baltimore, Maryland USA: STSci. Bibcode: 1997hst..prop.7393M.
- 1 2 3 4 5 6 7 John Krist and Richard Hook (June 2004). "The Tiny Tim User’s Guide, Version 6.3". Space Telescope Science Institute. Retrieved 2013-01-22. Unknown parameter
|pdf=
ignored (help) - 1 2 A. S. Wilson, J. A. Braatz, T. M. Heckman, J. H. Krolik, and G. K. Miley (December 20, 1993). "The Ionization Cones in the Seyfert Galaxy NGC 5728". The Astrophysical Journal Letters 419 (12): L61-4. doi:10.1086/187137.
- ↑ "Hubble Space Telescope, In: Wikipedia". San Francisco, California: Wikimedia Foundation, Inc. January 21, 2013. Retrieved 2013-01-22.
- ↑ "Wide Field Camera 3, In: Wikipedia". San Francisco, California: Wikimedia Foundation, Inc. January 15, 2013. Retrieved 2013-01-22.
- ↑ "filters - popular and hot telescope filters". Lumicon international. Retrieved 2010-11-22.
- ↑ "Astronomical filter, In: Wikipedia". San Francisco, California: Wikimedia Foundation, Inc. 25 January 2013. Retrieved 2013-01-25.
- 1 2 "Infrared detector, In: Wikipedia". San Francisco, California: Wikimedia Foundation, Inc. April 8, 2012. Retrieved 2012-06-19.
- ↑ Arline M. Melo, Mariano A. Kornberg, Pierre Kaufmann, Maria H. Piazzetta, Emílio C. Bortolucci, Maria B. Zakia, Otto H. Bauer, Albrecht Poglitsch, and Alexandre M. P. Alves da Silva (Nov 2008). "Metal mesh resonant filters for terahertz frequencies". Applied Optics 47 (32): 6064. doi:10.1364/AO.47.006064. PMID 19002231. http://www.opticsinfobase.org/abstract.cfm?URI=ao-47-32-6064.
- ↑ Ade, Peter A. R.; Pisano, Giampaolo; Tucker, Carole; Weaver, Samuel (Jul 2006). "A Review of Metal Mesh Filters". Millimeter and Submillimeter Detectors and Instrumentation for Astronomy III. Proceedings of the SPIE. 6275: 62750U. http://astrophysics.gsfc.nasa.gov/cosmology/spirit/tech_papers/Ade_filter_review.pdf.
- ↑ D. W. Porterfield, J. L. Hesler, R. Densing, E. R. Mueller, T. W. Crowe, and R. M. Weikle II (Sep 1994). "Resonant metal-mesh bandpass filters for the far infrared". Applied Optics 33 (25): 6046. doi:10.1364/AO.33.006046. PMID 20936018. http://www.opticsinfobase.org/ao/abstract.cfm?uri=ao-33-25-6046.
- ↑ Giampaolo Pisano, Giorgio Savini, Peter A. R. Ade, and Vic Haynes (2008). "Metal-mesh achromatic half-wave plate for use at submillimeter wavelengths". Applied Optics 47 (33): 6251–6256. doi:10.1364/AO.47.006251. PMID 19023391. http://www.opticsinfobase.org/ao/abstract.cfm?uri=ao-47-33-6251.
- ↑ "Metal-mesh optical filters, In: Wikipedia". San Francisco, California: Wikimedia Foundation, Inc. February 20, 2013. Retrieved 2013-05-25.
- ↑ "Coherer, In: Wikipedia". San Francisco, California: Wikimedia Foundation, Inc. April 22, 2013. Retrieved 2013-05-25.
- 1 2 3 4 5 "Cherenkov detector, In: Wikipedia". San Francisco, California: Wikimedia Foundation, Inc. May 14, 2013. Retrieved 2013-05-17.
- 1 2 3 "Ring-imaging Cherekov detector, In: Wikipedia". San Francisco, California: Wikimedia Foundation, Inc. April 15, 2013. Retrieved 2013-05-17.
- ↑ A.Augusto Alves Jr. et al (2008). "The LHCb Detector at the LHC". JINST 3 S08005.
- ↑ M.Adinolfi et al (2012). "Performance of the LHCb RICH detector at the LHC". http://arxiv.org/abs/arXiv:1211.6759.
- ↑ K. L. Brown, G. W. Tautfest (September 1956). "Faraday-Cup Monitors for High-Energy Electron Beams" (PDF). Review of Scientific Instruments 27 (9): 696–702. doi:10.1063/1.1715674. http://scitation.aip.org/getpdf/servlet/GetPDFServlet?filetype=pdf&id=RSINAK000027000009000696000001&idtype=cvips&prog=normal. Retrieved 2007-09-13.
- ↑ "Faraday cup, In: Wikipedia". San Francisco, California: Wikimedia Foundation, Inc. May 16, 2013. Retrieved 2013-05-25.
- ↑ "Gas detector, In: Wikipedia". San Francisco, California: Wikimedia Foundation, Inc. May 17, 2013. Retrieved 2013-05-26.
- ↑ Gustavo Alverio. "A Nanoparticle-based Hydrogen Microsensor". University of Central Florida. Retrieved 2008-10-21.
- ↑ D.R. Baselt. "Design and performance of a microcantilever-based hydrogen sensor". Sensors and Actuators B.
- ↑ Sumio Okuyama. Hydrogen Gas Sensing Using a Pd-Coated Cantilever. Japanese Journal of Applied Physics. http://jjap.jsap.jp/link?JJAP/39/3584/. Retrieved 2013-02-26.
- ↑ Jonas Henriksson. "Ultra-low power hydrogen sensing based on a palladium-coated nanomechanical beam resonator". Nanoscale Journal.
- ↑ "Hydrogen sensor, In: Wikipedia". San Francisco, California: Wikimedia Foundation, Inc. March 16, 2013. Retrieved 2013-05-26.
- ↑ "Liquid, In: Wikipedia". San Francisco, California: Wikimedia Foundation, Inc. May 25, 2013. Retrieved 2013-05-26.
- ↑ "Geophysics, In: Wikipedia". San Francisco, California: Wikimedia Foundation, Inc. October 18, 2012. Retrieved 2012-11-16.
- ↑ JHU/APL (January 30, 2008). "Mercury Shows Its True Colors". Baltimore, Maryland USA: JHU/APL. Retrieved 2013-04-01.
- ↑ Steven J. Ostro (October-December 1993). "Planetary radar astronomy". Reviews of Modern Physics 65 (4): 1235-79. doi:10.1103/RevModPhys.65.1235. http://rmp.aps.org/abstract/RMP/v65/i4/p1235_1. Retrieved 2012-02-09.
- ↑ "X-ray fluorescence, In: Wikipedia". San Francisco, California: Wikimedia Foundation, Inc. March 20, 2013. Retrieved 2013-05-12.
- ↑ P. Sonnentrucker, D. A. Neufeld, T. G. Phillips, M. Gerin, D. C. Lis, M. De Luca, J. R. Goicoechea, J. H. Black, T. A. Bell, F. Boulanger, J. Cernicharo, A. Coutens, E. Dartois, M . Kaźmierczak, P. Encrenaz, E. Falgarone, T. R. Geballe, T. Giesen, B. Godard, P. F. Goldsmith, C. Gry, H. Gupta, P. Hennebelle, E. Herbst, P. Hily-Blant, C. Joblin, R. Kołos, J. Krełowski, J. Martín-Pintado, K. M. Menten, R. Monje, B. Mookerjea, J. Pearson, M. Perault, C. M. Persson, R. Plume, M. Salez, S. Schlemmer, M. Schmidt, J. Stutzki, D.Teyssier, C. Vastel, S. Yu, E. Caux, R. Güsten, W. A. Hatch, T. Klein, I. Mehdi, P. Morris and J. S. Ward (October 1, 2010). "Detection of hydrogen fluoride absorption in diffuse molecular clouds with Herschel/HIFI: a ubiquitous tracer of molecular gas". Astronomy & Astrophysics 521: 5. doi:10.1051/0004-6361/201015082. http://arxiv.org/pdf/1007.2148.pdf. Retrieved 2013-01-17.
- ↑ M. A. Gurwell, E. A. Bergin, G. J. Melnick, M. L. N. Ashby, G. Chin, N. R. Erickson, P. F. Goldsmith, M. Harwit, J. E. Howe, S. C. Kleiner, D. G. Koch, D. A. Neufeld, B. M. Patten, R. Plume, R. Schieder, R. L. Snell, J. R. Stauffer, V. Tolls, Z. Wang, G. Winnewisser, and Y. F. Zhang (August 20, 2000). "Submillimeter Wave Astronomy Satellite Observations of the Martian Atmosphere: Temperature and Vertical Distribution of Water Vapor". The Astrophysical Journal 539 (2): L143-6. http://iopscience.iop.org/1538-4357/539/2/L143/pdf/1538-4357_539_2_L143.pdf. Retrieved 2012-08-04.
- 1 2 3 4 "Metal detector, In: Wikipedia". San Francisco, California: Wikimedia Foundation, Inc. May 13, 2013. Retrieved 2013-05-17.
- ↑ "Star Camera". NASA. 05/04. Archived from the original on July 21, 2011. Retrieved 25 May 2012.
- 1 2 "Big Bear Solar Observatory, In: Wikipedia". San Francisco, California: Wikimedia Foundation, Inc. October 31, 2012. Retrieved 2012-11-06.
- ↑ "Big Bear Solar Observatory - Causeway". Big Bear Solar Observatory. Retrieved 2012-01-15.
- ↑ R. Abbasi et al. (IceCube Collaboration) (2010). "Calibration and Characterization of the IceCube Photomultiplier Tube". Nuclear Instruments and Methods A 618: 139–152. doi:10.1016/j.nima.2010.03.102.
- ↑ R. Abbasi et al. (IceCube Collaboration) (2009). "The IceCube Data Acquisition System: Signal Capture, Digitization, and Timestamping". Nuclear Instruments and Methods A 601: 294–316. doi:10.1016/j.nima.2009.01.001.
- ↑ "IceCube Neutrino Observatory, In: Wikipedia". San Francisco, California: Wikimedia Foundation, Inc. August 10, 2012. Retrieved 2012-08-23.
- ↑ "ANTARES (telescope), In: Wikipedia". San Francisco, California: Wikimedia Foundation, Inc. July 4, 2012. Retrieved 2012-08-23.
- ↑ Heasarc (June 26, 2003). "OSO-5". Greenbelt, Maryland USA: NASA GSFC. Retrieved 2013-05-18.
- ↑ Niels Bohr (1985), "Rydberg's discovery of the spectral laws", in J. Kalckar, N. Bohr: Collected Works, 10, North-Holland Publ.
- ↑ "Hydrogen spectral series, In: Wikipedia". San Francisco, California: Wikimedia Foundation, Inc. May 2, 2012. Retrieved 2012-05-14.
- 1 2 "Superluminal motion, In: Wikipedia". San Francisco, California: Wikimedia Foundation, Inc. April 22, 2013. Retrieved 2013-05-26.
- ↑ Albert C. Thompson. X-Ray Data Booklet, Section 4-5 X-ray detectors. http://xdb.lbl.gov/Section4/Sec_4-5.pdf.
- 1 2 Leo, W. R. (1994). “Techniques for Nuclear and particle Physics Experiments”, 2nd edition, Springer, ISBN 354057280
- 1 2 3 "Scintillator, In: Wikipedia". San Francisco, California: Wikimedia Foundation, Inc. June 7, 2012. Retrieved 2012-06-19.
- ↑ "Scintillation counter, In: Wikipedia". San Francisco, California: Wikimedia Foundation, Inc. April 7, 2013. Retrieved 2013-05-17.
- ↑ Yousuke, I.; Daiki, S.; Hirohiko, K.; Nobuhiro, S.; Kenji, I. (2000). "Deterioration of pulse-shape discrimination in liquid organic scintillator at high energies". Nuclear Science Symposium Conference Record, Volume: 1 (IEEE) 1: 6/219–6/221 vol.1. doi:10.1109/NSSMIC.2000.949173. ISBN 0-7803-6503-8.
- ↑ Kawaguchi, N.; Yanagida, T.; Yokota, Y.; Watanabe, K.; Kamada, K.; Fukuda, K.; Suyama, T.; Yoshikawa, A. (2009). "Study of crystal growth and scintillation properties as a neutron detector of 2-inch diameter eu doped LiCaAlF6 single crystal". Nuclear Science Symposium Conference Record (NSS/MIC) (IEEE): 1493–1495. doi:10.1109/NSSMIC.2009.5402299. ISBN 978-1-4244-3961-4.
- ↑ Example crystal scintillator based neutron monitor.
- ↑ Bollinger, L.M.; Thomas, G.E.; Ginther, R.J. (1962). "Neutron Detection With Glass Scintillators". Nuclear Instruments and Methods 17: 97–116.
- ↑ Miyanaga, N.; Ohba, N.; Fujimoto, K. (1997). "Fiber scintillator/streak camera detector for burn history measurement in inertial confinement fusion experiment". Review of Scientific Instruments 68 (1): 621–623. doi:10.1063/1.1147667.
- ↑ G. F. Knoll (1999). Radiation Detection and Measurement, 3rd edition. Wiley. ISBN 978-0471073383.
- ↑ Mireshghi, A.; Cho, G.; Drewery, J.S.; Hong, W.S.; Jing, T.; Lee, H.; Kaplan, S.N.; Perez-Mendez, V. (1994). "High efficiency neutron sensitive amorphous silicon pixel detectors". Nuclear Science (IEEE) 41 (4 , Part: 1–2): 915–921. doi:10.1109/23.322831.
- ↑ Klaus Hinkelmann, Oscar Kempthorne (2008). Design and Analysis of Experiments, Volume I: Introduction to Experimental Design (2nd ed.). Wiley. ISBN 978-0-471-72756-9. http://books.google.com/?id=T3wWj2kVYZgC&printsec=frontcover.
- ↑ R. A. Bailey (2008). Design of comparative experiments. Cambridge University Press. ISBN 978-0-521-68357-9. http://www.cambridge.org/uk/catalogue/catalogue.asp?isbn=9780521683579.
- ↑ "Treatment and control groups, In: Wikipedia". San Francisco, California: Wikimedia Foundation, Inc. May 18, 2012. Retrieved 2012-05-31.
- ↑ "proof of concept, In: Wiktionary". San Francisco, California: Wikimedia Foundation, Inc. November 10, 2012. Retrieved 2013-01-13.
- ↑ Ginger Lehrman and Ian B Hogue, Sarah Palmer, Cheryl Jennings, Celsa A Spina, Ann Wiegand, Alan L Landay, Robert W Coombs, Douglas D Richman, John W Mellors, John M Coffin, Ronald J Bosch, David M Margolis (August 13, 2005). "Depletion of latent HIV-1 infection in vivo: a proof-of-concept study". Lancet 366 (9485): 549-55. doi:10.1016/S0140-6736(05)67098-5. http://www.ncbi.nlm.nih.gov/pmc/articles/PMC1894952/. Retrieved 2012-05-09.
- ↑ "Proof of concept, In: Wikipedia". San Francisco, California: Wikimedia Foundation, Inc. December 27, 2012. Retrieved 2013-01-13.
- 1 2 3 "prototype, In: Wiktionary". San Francisco, California: Wikimedia Foundation, Inc. December 8, 2013. Retrieved 2014-01-03.
- ↑ "field-test, In: Wiktionary". San Francisco, California: Wikimedia Foundation, Inc. August 5, 2012. Retrieved 2013-01-13.
- ↑ A. Liu; I. Gorton (March/April 2003). "Accelerating COTS middleware acquisition: the i-Mate process". Software, IEEE 20 (2): 72-9. doi:10.1109/MS.2003.1184171. http://cin.ufpe.br/~redis/intranet/bibliography/middleware/liu-cots03.pdf. Retrieved 2012-02-15.
- ↑ Rhea Wessel (January 25, 2008). "Cargo-Tracking System Combines RFID, Sensors, GSM and Satellite". RFID Journal: 1-2. http://www.rfidjournal.com/article/pdf/3870/1/1/rfidjournal-article3870.PDF. Retrieved 2012-02-15.
- ↑ P. Suresh, C. Rao, M.D. Annable and J.W. Jawitz (August 2000). E. Timothy Oppelt. ed. [http://www.afcee.af.mil/shared/media/document/AFD-071003-081.pdf#page=108 In Situ Flushing for Enhanced NAPL Site Remediation: Metrics for Performance Assessment, In: Abiotic In Situ Technologies for Groundwater Remediation Conference]. Cincinnati, Ohio: U.S. Environmental Protection Agency. pp. 105. http://www.afcee.af.mil/shared/media/document/AFD-071003-081.pdf#page=108. Retrieved 2012-02-15.
Further reading
- C. Grupen (June 28-July 10 1999). Physics of Particle Detection. 536. Istanbul: Dordrecht, D. Reidel Publishing Co.. pp. 3–34. doi:10.1063/1.1361756.
- "Radiation detectors". H. M. Stone Productions, Schloat. Tarrytown, N.Y., Prentice-Hall Media, 1972.
External links
- African Journals Online
- Bing Advanced search
- Google Books
- Google scholar Advanced Scholar Search
- How to Build a Cloud Chamber
- International Astronomical Union
- JSTOR
- Lycos search
- NASA/IPAC Extragalactic Database - NED
- NASA's National Space Science Data Center
- NCBI All Databases Search
- Office of Scientific & Technical Information
- PubChem Public Chemical Database
- Questia - The Online Library of Books and Journals
- SAGE journals online
- The SAO/NASA Astrophysics Data System
- Scirus for scientific information only advanced search
- SDSS Quick Look tool: SkyServer
- SIMBAD Astronomical Database
- Spacecraft Query at NASA.
- SpringerLink
- Taylor & Francis Online
- WikiDoc The Living Textbook of Medicine
- Wiley Online Library Advanced Search
- Yahoo Advanced Web Search
|
This is a research project at http://en.wikiversity.org
![]() |
Development status: this resource is experimental in nature. |
![]() |
Resource type: this resource is an article. |
![]() |
Resource type: this resource contains a lecture or lecture notes. |
![]() |
Subject classification: this is an astronomy resource. |
![]() |
Subject classification: this is a materials science resource. |
![]() |
Subject classification: this is a physics resource . |
![]() |
Subject classification: this is a technology resource . |