Radiation astronomy/Neutrals
< Radiation astronomy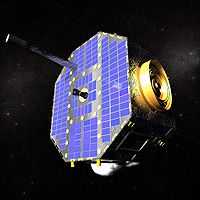
Neutrals astronomy is the astronomy of observing neutral atoms or molecules, their sources and apparent entities or objects of origin.
Strong forces
A "new type of neutron star model (Q stars) [is such that] high-density, electrically neutral baryonic matter is a coherent classical solution to an effective field theory of strong forces and is bound in the absence of gravity. [...] allows massive compact objects, [...] and has no macroscopic minimum mass."[1]
"Compact objects in astronomy are usually analyzed in terms of theoretical characteristics of neutron stars or black holes that are based upon calculations of equations of state for matter at very high densities. At such high densities, the effects of strong forces cannot be neglected. There are several conventional approaches to describing nuclear forces, all of which find that for a baryon number greater than ~250, a nucleus will become energetically unbound. High-density hadronic matter is not stable in these theories until there are enough baryons for gravitational binding to form a neutron star, typically with a minimum mass ≳ 0.1 M⊙ and maximum mass ≲ 3 M⊙."[1]
"Another possibility [called "baryon matter"] is that in the absence of gravity high-density baryonic matter is bound by purely strong forces. [...] nongravitationally bound bulk hadronic matter is consistent with nuclear physics data [...] and low-energy strong interaction data [...] The effective field theory approach has many successes in nuclear physics [...] suggesting that bulk hadronic matter is just as likely to be a correct description of matter at high densities as conventional, unbound hadronic matter."[1]
"The idea behind baryon matter is that a macroscopic state may exist in which a smaller effective baryon mass inside some region makes the state energetically favored over free particles. [...] This state will appear in the limit of large baryon number as an electrically neutral coherent bound state of neutrons, protons, and electrons in β-decay equilibrium."[1]
Neutrons
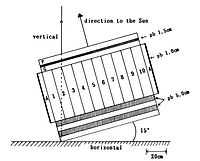
A "new detector to observe solar neutrons [has been in operation] since 1990 October 17 [...] at the Mount Norikura Cosmic Ray Laboratory (CRL) of [the] Institute for cosmic Ray Research, the University of Tokyo."[2]
"The solar neutron telescope [image at right] consists of 10 blocks of scintillator [...] and several lead plates which are used to place kinetic energies Tn of incoming particles into three bands (50-360 MeV, 280-500 MeV, and ≥ 390 MeV)."[2] The telescope is inclined to the direction of the Sun by 15°.[2] The plane area of the detector is 1.0 m2 and protected by lead plates (Pb) to eliminate gamma-ray and muon background from the side of the detector.[2] The anti-coincident counter (A) is used to reject the muons and gamma rays, coming from the side of the detector and the top scintillators.[2] (P) and (G) are used to identify the proton events and gamma rays.[2] The central scintillator blocks are optically separated into 10 units.[2]
"The horizontal scintillator just above the 10 vertical scintillators distinguishes neutral particles (neutrons) from the charged particles (mainly muons, protons and electrons)."[2]
Mesons
Single π0 production occurs "in neutral current neutrino interactions with water by a 1.3 GeV wide band neutrino beam."[3]
"The Gamma-Ray Spectrometer (GRS) on [Solar Maximum Mission] SMM has detected [...] at least two of the flares have spectral properties >40 MeV that require gamma rays from the decay of neutral pions. [Pion] production can occur early in the impulsive phase as defined by hard X-rays near 100 keV."[4]
Gamma-ray "emission matches remarkably well both the position and shape of the inner [supernova remnant] SNR shocked plasma. Furthermore, the gamma-ray spectrum shows a prominent peak near 1 GeV with a clear decrement at energies below a few hundreds of MeV as expected from neutral pion decay."[5]
X-rays
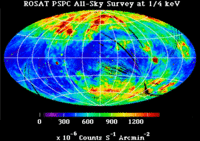
"By comparing the soft X-ray background with the distribution of neutral hydrogen, it is generally agreed that within the Milky Way disk, super soft X-rays are absorbed by this neutral hydrogen."[6]
The ROSAT image at the right is an Aitoff-Hammer equal-area map in galactic coordinates with the Galactic center in the middle of the 0.25 keV diffuse X-ray background.
Plasma objects
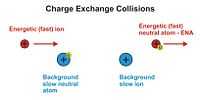
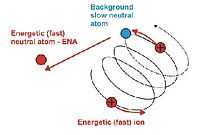
"In 1951, prior to the Space Age, the existence of energetic neutral hydrogen atoms (as high as 70 keV in energy) in space plasma was discovered."[8]
"ENA imaging permits study of the ways in which our entire plasma environment -- including the magnetopause, ring current, plasmasphere, auroral zones, plasma sheet, and the ionosphere -- reacts to the changing conditions of the solar wind (Williams, 1990)."[8]
Hydrogens
"Proton–hydrogen charge-exchange collisions [such as those shown at right] are often the most important process in space plasma because hydrogen is the most abundant constituent of both plasmas and background gases and hydrogen charge-exchange occurs at very high velocities involving little exchange of momentum."[9]
Sun
As stars are defined as luminous balls of plasma, the Sun may not qualify as its photosphere has a plasma concentration of approximately 10-4. The rest is composed of neutral atoms at about 5800 K.
Earth
"Energetic neutral atoms (ENA), emitted from the magnetosphere with energies of ∼50 keV, have been measured with solid-state detectors on the IMP 7/8 and ISEE 1 spacecraft. The ENA are produced when singly charged trapped ions collide with the exospheric neutral hydrogen geocorona and the energetic ions are neutralized by charge exchange."[10]
"The IMAGE mission ... High Energy Neutral Atom imager (HENA) ... images [ENAs] at energies between 10 and 60 keV/nucleon [to] reveal the distribution and the evolution of energetic [ions, including protons] as they are injected into the ring current during geomagnetic storms, drift about the Earth on both open and closed drift paths, and decay through charge exchange to pre‐storm levels."[11]
Moon
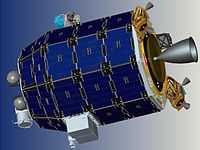
"The Lunar Atmosphere and Dust Environment Explorer (LADEE, at left) launched 07 September 2013 at 03:27 UT (06 September 11:27 EDT) on a Minotaur-V from Wallops Flight Facility. LADEE is designed to characterize the tenuous lunar atmosphere and dust environment from orbit. The scientific objectives of the mission are:(1) determine the global density, composition, and time variability of the fragile lunar atmosphere; and, (2) determine the size, charge, and spatial distribution of electrostatically transported dust grains and assess their likely effects on lunar exploration and lunar-based astronomy. Further objectives are to determine if the Apollo astronaut sightings of diffuse emission at 10s of km above the surface were Na glow or dust and document the dust impactor environment (size-frequency) to help guide design engineering for outpost and future robotic missions."[12]
"The orbiter will carry a Neutral Mass Spectrometer (NMS), an Ultraviolet/Visible Spectrometer (UVS), and a Lunar Dust Experiment (LDEX)."[12]
"The NMS is a quadrupole mass spectrometer designed ot detect species up to 150 amu and will look for CH4, S, O, Si, Kr, Xe, Fe, Al, Ti, Mg, OH, and H2O. The UVS will detect Al, Ca, Fe, K, Li, Na, Si, T, Ba, Mg, H2O, and O and will monitor the dust composition. The LDEX is an impact ionization dust detector designed to measure particles down to 0.3 microns at the spacecraft altitude. The LLCD is a test of a high data-rate optical (laser) communications system."[12]
Heliospheres
"The heliosphere is a bubble in space "blown" into the interstellar medium (the hydrogen and helium gas that permeates the galaxy) by the solar wind. Although electrically neutral atoms from interstellar volume can penetrate this bubble, virtually all of the material in the heliosphere emanates from the Sun itself."[13]
Interstellar medium
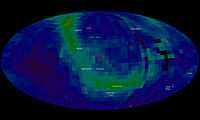
"In 2009, NASA's Interstellar Boundary Explorer (IBEX) mission science team constructed the first-ever all-sky map [at right] of the interactions occurring at the edge of the solar system, where the sun's influence diminishes and interacts with the interstellar medium. A 2013 paper provides a new explanation for a giant ribbon of energetic neutral atoms – shown here in light green and blue -- streaming in from that boundary."[14]
"[T]he boundary at the edge of our heliosphere where material streaming out from the sun interacts with the galactic material ... emits no light and no conventional telescope can see it. However, particles from inside the solar system bounce off this boundary and neutral atoms from that collision stream inward. Those particles can be observed by instruments on NASA’s Interstellar Boundary Explorer (IBEX). Since those atoms act as fingerprints for the boundary from which they came, IBEX can map that boundary in a way never before done. In 2009, IBEX saw something in that map that no one could explain: a vast ribbon dancing across this boundary that produced many more energetic neutral atoms than the surrounding areas."[14]
""What we are learning with IBEX is that the interaction between the sun's magnetic fields and the galactic magnetic field is much more complicated than we previously thought," says Eric Christian, the mission scientist for IBEX at NASA's Goddard Space Flight Center in Greenbelt, Md. "By modifying an earlier model, this paper provides the best explanation so far for the ribbon IBEX is seeing.""[14]
H I regions
"An H I region is an interstellar cloud composed of neutral atomic hydrogen (H I), in addition to the local abundance of helium and other elements."[15]
SIMBAD contains some 6,010 entries of the astronomical object type 'HI' (H I region).
"These regions are non-luminous, save for emission of the 21-cm (1,420 MHz) region spectral line. ... Mapping H I emissions with a radio telescope is a technique used for determining the structure of spiral galaxies."[15].
"The degree of ionization in an H I region is very small at around 10−4 (i.e. one particle in 10,000). The temperature of an H I region is about 100 K,[16] and it is usually considered as isothermal, except near an expanding H II region.[17]"[15].
For hydrogen, complete ionization "obviously reduces its cross section to zero, but ... the net effect of partial ionization of hydrogen on calculated absorption depends on whether or not observations of hydrogen [are] used to estimate the total gas. ... [A]t least 20 % of interstellar hydrogen at high galactic latitudes seems to be ionized".[18]
Cold neutral mediums
H I regions of the ISM contain the cold neutral medium (CNM). The CNM constitutes 1-5 % by volume of the ISM, ranges in size from 100-300 pc, has a temperature between 50 and 100 K, with an atom density of 20-50 atoms/cm3.[19] The CNM has hydrogen in the neutral atomic state and emits the 21 cm line.
Warm neutral mediums
The warm neutral medium (WNM) is 10-20 % of the ISM, ranges in size from 300-400 pc, temperature between 6000 and 10000 K, is composed of neutral atomic hydrogen, has a density of 0.2-0.5 atoms/cm3, and emits the hydrogen 21 cm line.[19]
Wolf-Rayet stars
"At the low density given by the spherically symmetric wind model (see Table 1), the dominant species in the gas are atomic ions while as the gas number density increases, the recombination of ions takes place and the gas composition is governed by neutral-phase chemistry, that is, the dominant species are neutral atoms and molecules although electrons and some ions are still present in relatively large amounts (for example, C+, O+ and He+)."[20]
Technology
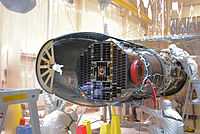
"The sensors on the IBEX spacecraft are able to detect energetic neutral atoms (ENAs) at a variety of energy levels."[21]
"The satellite's payload consists of two energetic neutral atom (ENA) imagers, IBEX-Hi and IBEX-Lo. Each of these sensors consists of a collimator that limits their fields-of-view, a conversion surface to convert neutral hydrogen and oxygen into ions, an electrostatic analyzer (ESA) to suppress ultraviolet light and to select ions of a specific energy range, and a detector to count particles and identify the type of each ion."[22]
"IBEX–Lo can detect particles with energies ranging from 10 electron–volts to 2,000 electron–volts (0.01 keV to 2 keV) in 8 separate energy bands. IBEX–Hi can detect particles with energies ranging from 300 electron–volts to 6,000 electron–volts (.3 keV to 6 keV) in 6 separate energy bands. ... Looking across the entire sky, interactions occurring at the edge of our Solar System produce ENAs at different energy levels and in different amounts, depending on the process."[21]
“The Submillimeter Wave Astronomy Satellite (SWAS) [is in] low Earth orbit ... to make targeted observations of giant molecular clouds and dark cloud cores. The focus of SWAS is five spectral lines: water (H2O), isotopic water (H218O), isotopic carbon monoxide (13CO), molecular oxygen (O2), and neutral carbon (C I).”[23]
See also
References
- 1 2 3 4 Safi Bahcall, Bryan W. Lynn, and Stephen B. Selipsky (October 10, 1990). "New Models for Neutron Stars". The Astrophysical Journal 362 (10): 251-5. doi:10.1086/169261. http://adsabs.harvard.edu/abs/1990ApJ...362..251B. Retrieved 2014-01-11.
- 1 2 3 4 5 6 7 8 Y. Muraki, K. Murakami, M. Miyazaki, K. Mitsui. S. Shibata, S. Sakakibara, T. Sakai, T. Takahashi, T. Yamada, and K. Yamaguchi (December 1, 1992). "Observation of solar neutrons associated with the large flare on 1991 June 4". The Astrophysical Journal 400 (2): L75-8. http://adsabs.harvard.edu/full/1992ApJ...400L..75M. Retrieved 2013-12-07.
- ↑ S. Nakayama, C. Mauger, M.H. Ahn, S. Aoki, Y. Ashie, H. Bhang, S. Boyd, D. Casper, J.H. Choi, S. Fukuda, Y. Fukuda, R. Gran, T. Hara, M. Hasegawa, T. Hasegawa, K. Hayashi, Y. Hayato, J. Hill, A.K. Ichikawa, A. Ikeda, T. Inagaki, T. Ishida, T. Ishii, M. Ishitsuka, Y. Itow, T. Iwashita, H.I. Jang, J.S. Jang, E.J. Jeon, K.K. Joo, C.K. Jung, T. Kajita, J. Kameda, K. Kaneyuki, I. Kato, E. Kearns, A. Kibayashi, D. Kielczewska, B.J. Kim, C.O. Kim, J.Y. Kim, S.B. Kim, K. Kobayashi, T. Kobayashi, Y. Koshio, W.R. Kropp, J.G. Learned, S.H. Lim, I.T. Lim, H. Maesaka, T. Maruyama, S. Matsuno, C. Mcgrew, A. Minamino, S. Mine, M. Miura, K. Miyano, T. Morita, S. Moriyama, M. Nakahata, K. Nakamura, I. Nakano, F. Nakata, T. Nakaya, T. Namba, R. Nambu, K. Nishikawa, S. Nishiyama, K .Nitta, S. Noda, Y. Obayashi, A. Okada, Y. Oyama, M.Y. Pac, H. Park, C. Saji, M. Sakuda, A. Sarrat, T. Sasaki, N. Sasao, K. Scholberg, M. Sekiguchi, E. Sharkey, M. Shiozawa, K.K. Shiraishi, M. Smy, H.W. Sobel, J.L. Stone, Y. Suga, L.R. Sulak, A. Suzuki, Y. Suzuki, Y. Takeuchi, N. Tamura, M. Tanaka, Y. Totsuka, S. Ueda, M.R. Vagins, C.W. Walter, W. Wang, R.J. Wilkes, S. Yamada, S. Yamamoto, C. Yanagisawa, H. Yokoyama, J. Yoo, M. Yoshida, and J. Zalipska (July 2005). "Measurement of single π0 production in neutral current neutrino interactions with water by a 1.3 GeV wide band muon neutrino beam". Physics Letters B 619 (3-4): 255-62. http://arxiv.org/pdf/hep-ex/0408134.pdf?origin=publication_detail. Retrieved 2014-03-22.
- ↑ Forrest, D. J., Vestrand, W. T., Chupp, E. L., Rieger, E., Cooper, J. F., & Share, G. H. (August 1985). Neutral Pion Production in Solar Flares, In: 19th International Cosmic Ray Conference. 4. NASA. pp. 146-9. Bibcode: 1985ICRC....4..146F. http://adsabs.harvard.edu/full/1985ICRC....4..146F. Retrieved 2014-10-01.
- ↑ A. Giuliani, M. Cardillo, M. Tavani, Y. Fukui, S. Yoshiike, K. Torii, G. Dubner, G. Castelletti, G. Barbiellini, A. Bulgarelli, P. Caraveo, E. Costa, P.W. Cattaneo, A. Chen, T. Contessi, E. Del Monte, I. Donnarumma, Y. Evangelista, M. Feroci, F. Gianotti, F. Lazzarotto, F. Lucarelli, F. Longo, M. Marisaldi, S. Mereghetti, L. Pacciani, A. Pellizzoni, G. Piano, P. Picozza, C. Pittori, G. Pucella, M. Rapisarda, A. Rappoldi, S. Sabatini, P. Soffitta, E. Striani, M. Trifoglio, A. Trois, S. Vercellone, F. Verrecchia, V. Vittorin, S. Colafrancesco, P. Giommi, and G. Bignami (1 December 2011). "Neutral Pion Emission from Accelerated Protons in the Supernova Remnant W44". The Astrophysical Journal Letters 742 (2): L30. doi:10.1088/2041-8205/742/2/L30. http://arxiv.org/pdf/1111.4868. Retrieved 2014-10-02.
- ↑ "Astrophysical X-ray source, In: Wikipedia". San Francisco, California: Wikimedia Foundation, Inc. November 9, 2012. Retrieved 2012-12-24.
- 1 2 Mike Gruntman. "Charge Exchange Diagrams". Energetic Neutral Atoms Tutorial. Retrieved 2009-10-27.
- 1 2 K. C. Hsieh and C. C. Curtis (1998). Imaging Space Plasma With Energetic Neutral Atoms Without Ionization, In: Measurement Techniques in Space Plasmas: Fields. Geophysical Monograph 103. American Geophysical Union. pp. 235-49. http://www.agu.org/books/gm/v103/GM103p0235/GM103p0235.pdf. Retrieved 2014-10-02.
- ↑ "Energetic neutral atom, In: Wikipedia". San Francisco, California: Wikimedia Foundation, Inc. August 4, 2012. Retrieved 2012-08-12.
- ↑ E. C. Roelof, D. G. Mitchell, D. J. Williams (1985). "Energetic neutral atoms (E ∼ 50 keV) from the ring current: IMP 7/8 and ISEE 1". Journal of Geophysical Research 90 (A11): 10,991-11,008. doi:10.1029/JA090iA11p10991. http://www.agu.org/pubs/crossref/1985/JA090iA11p10991.shtml. Retrieved 2012-08-12.
- ↑ D. G. Mitchell, K. C. Hsieh, C. C. Curtis, D. C. Hamilton, H. D. Voes, E. C, Roelof, P. C:son-Brandt (2001). "Imaging two geomagnetic storms in energetic neutral atoms". Geophysical Research Letters 28 (6): 1151-4. doi:10.1029/2000GL012395. http://www.agu.org/pubs/crossref/2001/2000GL012395.shtml. Retrieved 2012-08-12.
- 1 2 3 Richard C. Elphic, Sarah Noble, and P. Butler Hine III (September 7, 2013). "Lunar Atmosphere and Dust Environment Explorer (LADEE)". Washington, DC USA: National Space Science Data Center, NASA. Retrieved 2014-01-07.
- ↑ "Heliosphere, In: Wikipedia". San Francisco, California: Wikimedia Foundation, Inc. February 10, 2012. Retrieved 2012-02-10.
- 1 2 3 Karen C. Fox (February 5, 2013). "A Major Step Forward in Explaining the Ribbon in Space Discovered by NASA’s IBEX Mission". Greenbelt, MD USA: NASA's Goddard Space Flight Center. Retrieved 2013-02-06.
- 1 2 3 "H I region, In: Wikipedia". San Francisco, California: Wikimedia Foundation, Inc. April 8, 2012. Retrieved 2012-05-30.
- ↑ L. Spitzer, M. P. Savedoff (1950). "The Temperature of Interstellar Matter. III". The Astrophysical Journal 111: 593. doi:10.1086/145303.
- ↑ Savedoff MP, Greene J (November 1955). "Expanding H II region". Astrophysical Journal 122 (11): 477–87. doi:10.1086/146109.
- ↑ Robert Morrison and Dan McCammon (July 1983). "Interstellar photoelectric absorption cross sections, 0.03-10 keV". The Astrophysical Journal 270 (7): 119-22.
- 1 2 K. Ferriere (2001). "The Interstellar Environment of our Galaxy". Reviews of Modern Physics 73 (4): 1031–66. doi:10.1103/RevModPhys.73.1031.
- ↑ I. Cherchneff, Y.H. Le Teuff, P.M. Williams, and A.G.G.M. Tielens (May 2000). "Dust formation in carbon-rich Wolf-Rayet stars. I. Chemistry of small carbon clusters and silicon species". Astronomy and Astrophysics 357 (5): 572-80. http://adsabs.harvard.edu/cgi-bin/nph-data_query?bibcode=2000A%26A...357..572C&link_type=ARTICLE&db_key=AST&high=. Retrieved 2011-12-05.
- 1 2 Dave McComas and Lindsay Bartolone (May 10, 2012). "IBEX: Interstellar Boundary Explorer". San Antonio, Texas USA: NASA Southwest Research Institute. Retrieved 2012-08-11.
- ↑ "Interstellar Boundary Explorer, In: Wikipedia". San Francisco, California: Wikimedia Foundation, Inc. May 13, 2012. Retrieved 2012-08-11.
- ↑ "Submillimetre astronomy". Wikipedia (San Francisco, California: Wikimedia Foundation, Inc). June 2, 2012. http://en.wikipedia.org/wiki/Submillimetre_astronomy. Retrieved 2012-06-08.
External links
- African Journals Online
- Bing Advanced search
- Google Books
- Google scholar Advanced Scholar Search
- International Astronomical Union
- JSTOR
- Lycos search
- NASA/IPAC Extragalactic Database - NED
- NASA's National Space Science Data Center
- NCBI All Databases Search
- Office of Scientific & Technical Information
- PubChem Public Chemical Database
- Questia - The Online Library of Books and Journals
- SAGE journals online
- The SAO/NASA Astrophysics Data System
- Scirus for scientific information only advanced search
- SDSS Quick Look tool: SkyServer
- SIMBAD Astronomical Database
- SIMBAD Web interface, Harvard alternate
- Spacecraft Query at NASA.
- SpringerLink
- Taylor & Francis Online
- Universal coordinate converter
- Wiley Online Library Advanced Search
- Yahoo Advanced Web Search
This is a research project at http://en.wikiversity.org
![]() |
Educational level: this is a research resource. |
![]() |
Resource type: this resource is an article. |
![]() |
Resource type: this resource contains a lecture or lecture notes. |
![]() |
Subject classification: this is an astronomy resource. |