Quarks
| |
![]() | |
A proton, is composed of two up quarks and one down quark. One quark has a blue colour charge, one has a red colour charge, and the third has a green or yellow colour charge. (The color assignment of individual quarks is not important, only that all three colors are present.) |
- The following is modified from w:quark.
Quarks are subatomic particles that combine to form composite particles called hadrons, the most stable of which are protons and neutrons, the components of nuclides.[1] Due to a phenomenon known as color confinement, quarks are never found in isolation; they can only be found within hadrons.[2][3] For this reason, much of what is known about quarks has been drawn from observations of the hadrons themselves.
There are six types of quarks, known as flavors: up, down, charm, strange, top, and bottom.[4] Up and down quarks have the lowest masses of all quarks. The heavier quarks rapidly change into up and down quarks through a process of particle decay: the transformation from a higher mass state to a lower mass state. Because of this, up and down quarks are generally stable and the most common in the universe, whereas charm, strange, top, and bottom quarks can only be produced in high energy collisions (such as those involving cosmic rays and in particle accelerators).
Quarks have various intrinsic properties, including electric charge, color charge, spin, and mass. Quarks are the only elementary particles in the Standard Model of particle physics to experience all four fundamental interactions, also known as fundamental forces (electromagnetism, gravitation, strong interaction, and weak interaction), as well as the only known particles whose electric charges are not integer multiples of the elementary charge. For every quark flavor there is a corresponding type of antiparticle, known as antiquark, that differs from the quark only in that some of its properties have equal magnitude but opposite sign.
| |
Types: | up, down, strange, charm, top, and bottom |
Generations: | 1st, 2nd, 3rd |
Interactions: | Electromagnetism, Gravitation, Strong, Weak |
Symbols: | u, d, s, c, t, b |
Antiparticles: | u, d, s, c, t, b |
Theorized: | Murray Gell-Mann (1964) George Zweig (1964) |
Discovered: | SLAC (~1968) |
Spin: | 1⁄2 |
Electric charge: | +2⁄3 e, −1⁄3 e |
Color charge: | Yes |
Particle statistics: | Fermionic |
The quark model was independently proposed by physicists Murray Gell-Mann and George Zweig in 1964.[5] Quarks were introduced as parts of an ordering scheme for hadrons, and there was little evidence for their physical existence until deep inelastic scattering experiments at SLAC in 1968.[6][7] All six flavors of quark have since been observed in accelerator experiments; the top quark, first observed at Fermilab in 1995, was the last to be discovered.[5]
Classification
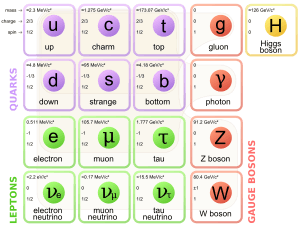
The Standard Model is the theoretical framework describing all the currently known elementary particles, as well as the unobserved[nb 1] Higgs boson.[8] This model contains six flavors of quarks (q), named up (u), down (d), charm (c), strange (s), top (t), and bottom (b).[4] Antiparticles of quarks are called antiquarks, and are denoted by a bar over the symbol for the corresponding quark, such as u for an up antiquark. As with antimatter in general, antiquarks have the same mass, mean lifetime, and spin as their respective quarks, but the electric charge and other charges have the opposite sign.[9]
Quarks are spin-1⁄2 particles, implying that they are fermions according to the spin-statistics theorem. They are subject to the Pauli exclusion principle, which states that no two identical fermions can simultaneously occupy the same quantum state. This is in contrast to bosons (particles with integer spin), of which any number can be in the same state.[10] Unlike leptons, quarks possess color charge, which causes them to engage in the strong interaction. The resulting attraction between different quarks causes the formation of composite particles known as hadrons (see "Strong interaction and color charge" below).
The quarks which determine the quantum numbers of hadrons are called valence quarks; apart from these, any hadron may contain an indefinite number of virtual (or sea) quarks, antiquarks, and gluons which do not influence its quantum numbers.[11] There are two families of hadrons: baryons, with three valence quarks, and mesons, with a valence quark and an antiquark.[12] The most common baryons are the proton and the neutron, the building blocks of the atomic nucleus.[13] A great number of hadrons are known (see list of baryons and list of mesons), most of them differentiated by their quark content and the properties these constituent quarks confer. The existence of "exotic" hadrons with more valence quarks, such as tetraquarks (qqqq) and pentaquarks (qqqqq), has been conjectured[14] but not proven.[nb 2][14][15]
Elementary fermions are grouped into three generations, each comprising two leptons and two quarks. The first generation includes up and down quarks, the second charm and strange quarks, and the third top and bottom quarks. All searches for a fourth generation of quarks and other elementary fermions have failed,[16] and there is strong indirect evidence that no more than three generations exist.[nb 3][17] Particles in higher generations generally have greater mass and lesser stability, causing them to decay into lower-generation particles by means of weak interactions. Only first-generation (up and down) quarks occur commonly in nature. Heavier quarks can only be created in high-energy collisions (such as in those involving cosmic rays), and decay quickly; however, they are thought to have been present during the first fractions of a second after the Big Bang, when the universe was in an extremely hot and dense phase (the quark epoch). Studies of heavier quarks are conducted in artificially created conditions, such as in particle accelerators.[18]
Having electric charge, mass, color charge, and flavor, quarks are the only known elementary particles that engage in all four fundamental interactions of contemporary physics: electromagnetism, gravitation, strong interaction, and weak interaction.[13] Gravitation is too weak to be relevant to individual particle interactions except at extremes of energy (Planck energy) and distance scales (Planck distance). However, since no successful quantum theory of gravity exists, gravitation is not described by the Standard Model.
See the properties of quarks for a more complete overview of the six quark flavors' properties.
See also
- History of quarks
- Properties of quarks
- Interacting quarks
- Gluon
- Color–flavor locking
- Model of quark quasiparticles
- Quarkonium – Mesons made of a quark and antiquark of the same flavor
- Preons – Hypothetical particles which were once postulated to be subcomponents of quarks and leptons
- Quark–lepton complementarity – Possible fundamental relation between quarks and leptons
- Quark star – A hypothetical degenerate neutron star with extreme density
- w:Book: Quarks
- w:Book: Hadronic Matter
- w:Book: Particles of the Standard Model
Notes
- ↑ As of Aug 2010.
- ↑ Several research groups claimed to have proven the existence of tetraquarks and pentaquarks in the early 2000s. While the status of tetraquarks is still under debate, all known pentaquark candidates have since been established as non-existent.
- ↑ The main evidence is based on the resonance width of the Z0
boson, which constrains the 4th generation neutrino to have a mass greater than ~45 GeV/c2. This would be highly contrasting with the other three generations' neutrinos, whose masses cannot exceed 2 MeV/c2.
References
- ↑ "Quark (subatomic particle)". Encyclopædia Britannica. Retrieved 2008-06-29.
- ↑ R. Nave. "Confinement of Quarks". HyperPhysics. Georgia State University, Department of Physics and Astronomy. Retrieved 2008-06-29.
- ↑ R. Nave. "Bag Model of Quark Confinement". HyperPhysics. Georgia State University, Department of Physics and Astronomy. Retrieved 2008-06-29.
- 1 2 R. Nave. "Quarks". HyperPhysics. Georgia State University, Department of Physics and Astronomy. Retrieved 2008-06-29.
- 1 2 B. Carithers, P. Grannis (1995). "Discovery of the Top Quark" (PDF). Beam Line (SLAC) 25 (3): 4–16. http://www.slac.stanford.edu/pubs/beamline/25/3/25-3-carithers.pdf. Retrieved 2008-09-23.
- ↑ E.D. Bloom et al. (1969). "High-Energy Inelastic e–p Scattering at 6° and 10°". Physical Review Letters 23 (16): 930–934. doi:10.1103/PhysRevLett.23.930.
- ↑ M. Breidenbach et al. (1969). "Observed Behavior of Highly Inelastic Electron–Proton Scattering". Physical Review Letters 23 (16): 935–939. doi:10.1103/PhysRevLett.23.935.
- ↑ C. Amsler et al. (Particle Data Group) (2008). "Higgs Bosons: Theory and Searches". Physics Letters B 667 (1): 1–1340. doi:10.1016/j.physletb.2008.07.018. http://pdg.lbl.gov/2009/reviews/rpp2009-rev-higgs-boson.pdf.
- ↑ S.S.M. Wong (1998). Introductory Nuclear Physics (2nd ed.). Wiley Interscience. p. 30. ISBN 0-471-23973-9.
- ↑ K.A. Peacock (2008). The Quantum Revolution. Greenwood Publishing Group. p. 125. ISBN 031333448X.
- ↑ B. Povh, C. Scholz, K. Rith, F. Zetsche (2008). Particles and Nuclei. Springer. p. 98. ISBN 3540793674.
- ↑ Section 6.1. in P.C.W. Davies (1979). The Forces of Nature. Cambridge University Press. ISBN 052122523X.
- 1 2 M. Munowitz (2005). Knowing. Oxford University Press. p. 35. ISBN 0195167376.
- 1 2 W.-M. Yao et al. (Particle Data Group) (2006). "Review of Particle Physics: Pentaquark Update". Journal of Physics G 33 (1): 1–1232. doi:10.1088/0954-3899/33/1/001. http://pdg.lbl.gov/2006/reviews/theta_b152.pdf.
- ↑ C. Amsler et al. (Particle Data Group) (2008). "Review of Particle Physics: Pentaquarks". Physics Letters B 667 (1): 1–1340. doi:10.1016/j.physletb.2008.07.018. http://pdg.lbl.gov/2008/reviews/pentaquarks_b801.pdf.
C. Amsler et al. (Particle Data Group) (2008). "Review of Particle Physics: New Charmonium-Like States". Physics Letters B 667 (1): 1–1340. doi:10.1016/j.physletb.2008.07.018. http://pdg.lbl.gov/2008/reviews/rpp2008-rev-new-charmonium-like-states.pdf.
E.V. Shuryak (2004). The QCD Vacuum, Hadrons and Superdense Matter. World Scientific. p. 59. ISBN 9812385746. - ↑ C. Amsler et al. (Particle Data Group) (2008). "Review of Particle Physics: b′ (4th Generation) Quarks, Searches for". Physics Letters B 667 (1): 1–1340. doi:10.1016/j.physletb.2008.07.018. http://pdg.lbl.gov/2008/listings/q008.pdf.
C. Amsler et al. (Particle Data Group) (2008). "Review of Particle Physics: t′ (4th Generation) Quarks, Searches for". Physics Letters B 667 (1): 1–1340. doi:10.1016/j.physletb.2008.07.018. http://pdg.lbl.gov/2008/listings/q009.pdf. - ↑ D. Decamp (1989). "Determination of the number of light neutrino species". Physics Letters B 231 (4): 519. doi:10.1016/0370-2693(89)90704-1.
A. Fisher (1991). "Searching for the Beginning of Time: Cosmic Connection". Popular Science 238 (4): 70. http://books.google.com/?id=eyPfgGGTfGgC&pg=PA70&dq=quarks+no+more+than+three+generations.
J.D. Barrow (1997) [1994]. "The Singularity and Other Problems". The Origin of the Universe (Reprint ed.). Basic Books. ISBN 978-0465053148. - ↑ D.H. Perkins (2003). Particle Astrophysics. Oxford University Press. p. 4. ISBN 0198509529.
Further reading
- D.J. Griffiths (2008). Introduction to Elementary Particles (2nd ed.). Wiley–VCH. ISBN 3527406018.
- I.S. Hughes (1985). Elementary particles (2nd ed.). Cambridge University Press. ISBN 0-521-26092-2.
- R. Oerter (2005). The Theory of Almost Everything: The Standard Model, the Unsung Triumph of Modern Physics. Pi Press. ISBN 0132366789.
- A. Pickering (1984). Constructing Quarks: A Sociological History of Particle Physics. The University of Chicago Press. ISBN 0-226-66799-5.
- B. Povh (1995). Particles and Nuclei: An Introduction to the Physical Concepts. Springer–Verlag. ISBN 0-387-59439-6.
- M. Riordan (1987). The Hunting of the Quark: A true story of modern physics. Simon & Schuster. ISBN 0-671-64884-5.
- B.A. Schumm (2004). Deep Down Things: The Breathtaking Beauty of Particle Physics. Johns Hopkins University Press. ISBN 0-8018-7971-X.
External links
- 1969 Physics Nobel Prize lecture by Murray Gell-Mann
- 1976 Physics Nobel Prize lecture by Burton Richter
- 1976 Physics Nobel Prize lecture by Samuel C.C. Ting
- 2008 Physics Nobel Prize lecture by Makoto Kobayashi
- 2008 Physics Nobel Prize lecture by Toshihide Maskawa
- The Top Quark And The Higgs Particle by T.A. Heppenheimer – A description of CERN's experiment to count the families of quarks.
Template:Particles Template:Composition Template:Featured article