Natural electric field of the Earth
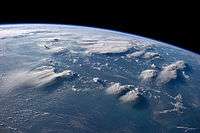
The natural electric field of the Earth refers to the planet Earth having a natural direct current (DC) electric field or potential gradient from the ground upwards to the ionosphere. The static fair-weather electric field in the atmosphere is ~150 volts per meter (V/m) near the Earth's surface, but it drops exponentially with height to under 1 V/m at 30 km altitude, as the conductivity of the atmosphere increases.
"In this image [on the right], late afternoon sunlight casts long shadows from thunderhead anvils down onto southern Borneo. Near the horizon (image top center), more than 1000 kilometers away from the space station, storm formation is assisted by air currents rising over the central mountains of Borneo."[1]
"Winds usually blow in different directions at different altitudes. At the time of this photo, high-altitude winds were clearly sweeping the tops off the many tallest thunderclouds, generating long anvils of diffuse cirrus plumes that trail south. At lower levels of the atmosphere, “streets” of white dots—fair-weather cumulus clouds—are aligned with west-moving winds. Small smoke plumes from forest fires in Borneo are also aligned west."[1]
Natural electric fields
The Earth is negatively charged, carrying 500,000 Coulombs (C) of electric charge (500 kC),[2] and is at 300,000 volts (V), 300 kV,[3] relative to the positively charged ionosphere. There is a constant flow of electricity, at around 1350 amperes (A) [approximately 1100 A][3], and resistance of the Earth's atmosphere is around 220 Ohms.[3] This gives a power output of around 400 megawatts (MW), which is ultimately regenerated by the power of the Sun that affects the ionosphere, as well as the troposphere, causing thunderstorms. The electrical energy stored in the Earth's atmosphere is around 150 gigajoules (GJ).
The Earth-ionosphere system acts as a giant capacitor, of capacity 1.8 Farads.
The Earth's surface carries around -1 nC of electric charge per square meter.
Electrospheres
The "electrosphere [of the Earth is] at about 60 km of altitude".[2]
Def. a "restricted region of the magnetosphere actually occupied by plasma"[4] is called an electrosphere.
For an aligned rotator, "Rotation induces a large pole-to-equator potential difference [...] If the work function at the surface were huge, a surface charge would appear on the sphere, in order to keep E·B = 0 in the interior. [...] charged rings [...] are released from the surface, one at a time. If [a Jupiter] choice of rotation vs field is made [...], negative particles come from the poles, positive from the equatorial zone. Each ring has the same charge. A ring released follows the magnetic field lines until it reaches a local E·B = 0 point. Positive rings from the equatorial region are therefore trapped and fill up an equatorial torus. Negative rings from the pole fly up along the field lines but do not escape if the total system charge is positive. After each new ring is released, the positions of all the others are shifted to new E·B = 0 points. Ultimately one reaches the point where there is not enough surface charge left to scrape together to make another ring of either charge [...] leaving behind a dome of negative charge and a torus of positive charge." These restricted regions are the electrosphere.[4]
Atmospheres
Below "approximately 50 km, conductivity [in the atmosphere] is mainly due to the presence of ions created by both cosmic rays and the Earth’s natural radioactivity."[2]
"Above 60 km, however, free electrons are the main contributors to this conductivity."[2]
"At surface level, the Earth’s natural radioactivity and cosmic rays play an equal role in creating these ions; at altitudes above 1 km, however, cosmic rays are mainly responsible for ion production in the Earth’s atmosphere."[2]
"The electrical conductivity of the air at sea level is about 10−14 Siemens/m (1 Siemens = 1/ohm) and increases rapidly with altitude; at 35 km, where the air density is only 1% of that at the surface, conductivity is about 10−11 S/m. About approximately 80 km, however, the conductivity of the atmosphere becomes anisotropic due to the influence of the Earth’s magnetic field and the diurnal variations of the solar photoionization processes."[2]
"The magnitude of [the natural electric] field decreases with altitude; at 10 km it has a value of about 3% of that at the surface, whereas at 30 km it is about 300 mV/m and 1 μV/m at about 85 km (Rakov and Uman, 2003)."[2]
The "value of the electrical field intensity, 3×106 V/m, [is] for electrical breakdown between two parallel plane electrodes at sea level in dry air (Rakov and Uman, 2003)."[2]
Fair weather
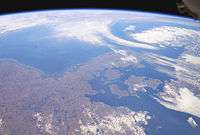
"This astronaut photograph [on the right], taken on February 26, 2003, is centered on the Kingdom of Denmark. The entire region is overlain with deposits of Pleistocene glaciers."[5]
"Taking advantage of remarkably fair weather over north central Europe for the time of year, the crew of the International Space Station took this panoramic view that extends from the North Sea coast of the Netherlands on the left to the Baltic Sea shores of Sweden on the right. The late-winter landscape has little snow cover except over northeastern Germany, Sweden, and the rugged mountains of Norway. Such images, composed by astronauts, provide unique, synoptic perspectives of the Earth's geography and natural processes."[5]
"Because of the Earth’s weak conducting capacity, a fair-weather current of about 2 × 10−12 A exists between the shells of this capacitor."[2]
"In fair weather conditions, the surface of the Earth is negatively charged and the electric field in the atmosphere varies typically between 100 and 150 V/m."[6]
"The Amazonian forest of about 5 x 106 km2, could, temporarily, conduct upward roughly 4 x 104 A, in the extreme – and admittedly somewhat unrealistic – case of all the transient signals being simultaneous on all the trees."[6]
"It is known that the earth is negatively charged and that there is a difference of potential between the earth's surface and a layer of the upper atmosphere of the order of 3 x 105 volts in fine weather; therefore any body rising from the earth's surface to this upper layer of the atmosphere will carry a negative charge at a potential of approximately 3 x 105 volts to the surrounding air, assuming of course that it has lost no charge by dissipation."[7]
"The electric field at the ground is an easily measurable quantity that has been measured at various locations. During fair weather the variation is small and the strength is ~ 100 V/m. However, the electric field on the ground can vary from -200 to 400 V/m if fog and haze ... In fair weather the ground electric field variations are due to changes in columnar resistance, ionospheric potential, and local conductivity".[8]
See also
References
- 1 2 M. Justin Wilkinson, Jacobs and Michael Trenchard (5 August 2014). "Thunderstorms over Borneo". Washington, DC USA: NASA. Retrieved 2016-01-06.
- 1 2 3 4 5 6 7 8 9 Lorenzo Labrador (April 5, 2005). "Sensitivity of Tropospheric Chemistry to the Source of NOx from Lightning: Simulations with the Global 3D Chemistry-Transport Model MATCH-MPIC". Heidelberg, Germany: University of Heidelberg. Retrieved 2014-12-20.
- 1 2 C Polk (1969). Samuel C. Coroniti, J. Hughes. ed. Relation of ELF Noise and Schumann Resonances to Thunderstorm Activity, In: Planetary Electrodynamics. 150 Fifth Avenue, New York, NY USA: Gordon and Breach Science Publishers. pp. 55-83. ISBN 0677136005, 9780677136004. https://books.google.com/books?id=wDXzhyskXOgC&printsec=frontcover&hl=en&sa=X&ei=1zeMVLYFh_nJBPHagdAN&ved=0CBQQ6AEwAA#v=onepage&f=false. Retrieved 2014-12-13.
- 1 2 F. Curtis Michel (February 1985). "Non neutral plasmas in the laboratory and astrophysics". Proceedings of the Astronomical Society of Australia 6 (2): 127-9. http://adsabs.harvard.edu/cgi-bin/nph-data_query?bibcode=1985PASAu...6..127M&link_type=ARTICLE&db_key=AST&high=555927d52a21583. Retrieved 2016-01-06.
- 1 2 Yvette Smith (26 February 2003). "A Danish Perspective". Washington, DC USA: NASA. Retrieved 2016-01-06.
- 1 2 Jean-Louis Le Mouël, Dominique Gibert, Jean-Paul Poirier (2010). "On transient electric potential variations in a standing tree and atmospheric electricity". Comptes Rendus Geoscience 342: 95-9. http://www.ipgp.fr/~gibert/PDF_Files/60.pdf. Retrieved 2014-12-13.
- ↑ B.L. Goodlet (February 1938). "The author's reply to the discussions on Lightning at Glasgow, Newcastle, Belfast, Manchester, Birmingham, Loughborough, Bristol and Middlesbrough". Journal of the Institution of Electrical Engineers 82 (494): 211-3. doi:10.1049/jiee-1.1938.0030. http://ieeexplore.ieee.org/xpls/abs_all.jsp?arnumber=5317467. Retrieved 2014-12-13.
- ↑ Eileen K. Stansbery (March 1989). A global model of thunderstorm electricity and the prediction of whistler duct formation. Houston, Texas USA: Rice University. pp. 174. http://scholarship.rice.edu/bitstream/handle/1911/16298/9012871.PDF?sequence=1. Retrieved 2015-01-03.
Further reading
- C Polk (1969). Samuel C. Coroniti, J. Hughes. ed. Relation of ELF Noise and Schumann Resonances to Thunderstorm Activity, In: Planetary Electrodynamics. 150 Fifth Avenue, New York, NY USA: Gordon and Breach Science Publishers. pp. 55-83. ISBN 0677136005, 9780677136004. https://books.google.com/books?id=wDXzhyskXOgC&printsec=frontcover&hl=en&sa=X&ei=1zeMVLYFh_nJBPHagdAN&ved=0CBQQ6AEwAA#v=onepage&f=false. Retrieved 2014-12-13.
External links
|
This is a research project at http://en.wikiversity.org
![]() |
Development status: this resource is experimental in nature. |
![]() |
Educational level: this is a research resource. |
![]() |
Resource type: this resource is an article. |
![]() |
Resource type: this resource contains a lecture or lecture notes. |
![]() |
Subject classification: this is a Geology resource. |