Mining geology
Exploration for, discovery of, and removal of valuable natural resources from the Earth is mining geology.
Geology
Geology is often used to determine how to acquire the resource from its natural and sometimes dangerous location.
Coal gas
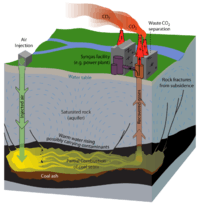
"Underground Coal Gasification (UCG) involves igniting coal in the ground, then collecting and using the gases that result from its partial combustion. Although the idea dates back over a century, very few UCG plants have ever been built. Underground gasification could potentially allow the use of coal that is currently uneconomical to mine. Underground gasification eliminates the need for strip mining and transportation of coal, as well as potentially making carbon capture and sequestration more practical. However, UCG produces large amounts of CO2, and the coal combustion wastes that are left behind can leach pollutants into nearby groundwater, and have caused major contamination in UCG pilot projects."[1]
"The form of UCG used in most modern projects consists of drilling two wells into a coal seam. Air or oxygen is injected into one well and a controlled combustion reaction is started in the seam itself - a more-controlled version of a natural coal seam fire. Gases are collected through the second well and are separated in a facility at the surface. This process produces primarily hydrogen and CO2, with lesser amounts of carbon monoxide, methane, and trace amounts of other gases."[1]
"These gases are then burned to produce energy, as in a natural gas plant. Hydrogen is the primary energy-containing gas in the mix. The combination of carbon monoxide and hydrogen is called syngas and can be combusted directly to produce heat or can be liquefied and refined in processes similar to that described for coal-to-liquids. Although CO2 is one of the major products of UCG, it is simply a waste product and does not contain any recoverable energy. Because the combustion occurs underground, it heats the surrounding rock. This portion of the heat is not accessible for industrial use, therefore UCG burns more coal (per unit energy produced) than would be required if it was first mined. However, conventional mining and transport of coal also requires significant energy and have associated releases of greenhouse gases."[1]
Environmental impacts
"Compared to conventional coal-fired power, underground gasification can greatly reduce the impacts associated with coal mining, coal dust, and the emissions of sulfur dioxide and nitrous oxides (responsible for acid rain and smog). However, UCG has several major environmental costs: contamination of ground water, subsidence of the overlying terrain, and global warming impacts. There is also a potential concern with the spread of underground coal seam fires, although this has not been observed in any trials to date."[1]
"In underground gasification, there is no need for above ground disposal of coal combustion wastes such as coal ash. However, these pollutants are left behind in the coal seam, where they can leach out into surrounding groundwater. Groundwater contamination has been a major problem in almost all pilot UCG projects, most most well-documented in the Hoe Creek project managed by Lawrence Livermore Laboratory. The combustion of coal traps a variety of toxic substances such as mercury, phenol, and benzene into the former coal seam. Additionally, the solubility of heavy metals in water can be increased if the coal seam is fully oxidized. Pollutants can leach out through the surrounding rock, or be taken up by water that enters the chamber. The Chinchilla pilot project attempted to address this problem by maintaining negative pressure inside the coal combustion chamber to limit the outflow of containments. However, the technology to prevent contamination is in its infancy, and groundwater pollution remains the single biggest concern with UCG. Groundwater contamination is particularly worrisome since the pollution source deep underground is inaccessible and permanent. Problems may crop up at any time during or after the project (including decades or centuries later) and cleanup will be difficult or impossible. Waste remaining underground presents a problem into the indefinite future, making eventual leakage likely."[1]
Porphyry copper metallogenesis
"Although the tectonic uplift of the 3000 m to 5000 m high range has generally been assumed to be mostly Miocene in age, field relationships suggest that the Domeyko Fault System and tectonic uplift were active as early as the Eocene, coinciding with porphyry copper emplacement between 41 Ma and 30 Ma. Apatite fission track (FT) thermochronology provides both age data and a time-temperature history for rocks since they cooled below a temperature of ca. 125 degrees C (equivalent to a depth of 4 km to 5 km under normal geothermal gradients) on their way to the surface during exhumation, or after a heating event."[2]
"Apatite FT data from the Paleozoic crystalline basement of the Domeyko Cordillera indicate that at least 4 km to 5 km of rocks were eroded during exhumation of this tectonic block between ca. 50 Ma to 30 Ma (Middle Eocene to Early Oligocene), a time that immediately precedes and overlaps with the emplacement of giant porphyry copper deposits. The FT data constrain the age and duration of a period of crustal thickening and extensive erosion known as the Incaic compression, an event recognized in the Andes of Chile and Peru."[2]
Nickels
.jpg)
The Talvivaara mine in Sotkamo, Finland, in the image on the right, extracts nickel from the Earth.
Research
Hypothesis:
- Ultimately, the best and final prospecting is on the ground by foot with detectors.
Control groups
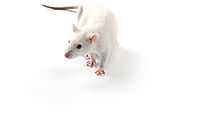
The findings demonstrate a statistically systematic change from the status quo or the control group.
“In the design of experiments, treatments [or special properties or characteristics] are applied to [or observed in] experimental units in the treatment group(s).[3] In comparative experiments, members of the complementary group, the control group, receive either no treatment or a standard treatment.[4]"[5]
Proof of concept
Def. a “short and/or incomplete realization of a certain method or idea to demonstrate its feasibility"[6] is called a proof of concept.
Def. evidence that demonstrates that a concept is possible is called proof of concept.
The proof-of-concept structure consists of
- background,
- procedures,
- findings, and
- interpretation.[7]
Proof of technology
"[T]he objective of a proof of technology is to determine the solution to some technical problem, such as how two systems might be integrated or that a certain throughput can be achieved with a given configuration."[8]
Def.
- "[a]n original object or form which is a basis for other objects, forms, or for its models and generalizations",[9]
- "[a]n early sample or model built to test a concept or process",[9] or
- "[a]n instance of a category or a concept that combines its most representative attributes"[9] is called a prototype.
Def. "[t]o test something using the conditions that it was designed to operate under, especially out in the real world instead of in a laboratory or workshop"[10] is called "field-test", or a field test.
A "proof-of-technology prototype ... typically implements one critical scenario to exercise or stress the highest-priority requirements."[11]
"[A] proof-of-technology test demonstrates the system can be used"[12].
"The strongest proof of technology performance is based on consistency among multiple lines of evidence, all pointing to similar levels of risk reduction."[13]
See also
References
- 1 2 3 4 5 David Coil, Erin McKittrick, Bretwood Higman, and Ground Truth Trekking (3 October 2014). "Underground Coal Gasification (UCG)". Ground Truth Trekking. Retrieved 2015-01-11.
- 1 2 Victor Maksaev and Marcos Zentilli (April 1999). "Fission track thermochronology of the Domeyko Cordillera, northern Chile; implications for Andean tectonics and porphyry copper metallogenesis". Exploration and Mining Geology 8 (1-2): 65-89. http://emg.geoscienceworld.org/content/8/1-2/65.short. Retrieved 2015-09-12.
- ↑ Klaus Hinkelmann, Oscar Kempthorne (2008). Design and Analysis of Experiments, Volume I: Introduction to Experimental Design (2nd ed.). Wiley. ISBN 978-0-471-72756-9. http://books.google.com/?id=T3wWj2kVYZgC&printsec=frontcover.
- ↑ R. A. Bailey (2008). Design of comparative experiments. Cambridge University Press. ISBN 978-0-521-68357-9. http://www.cambridge.org/uk/catalogue/catalogue.asp?isbn=9780521683579.
- ↑ "Treatment and control groups, In: Wikipedia". San Francisco, California: Wikimedia Foundation, Inc. May 18, 2012. Retrieved 2012-05-31.
- ↑ "proof of concept, In: Wiktionary". San Francisco, California: Wikimedia Foundation, Inc. November 10, 2012. Retrieved 2013-01-13.
- ↑ Ginger Lehrman and Ian B Hogue, Sarah Palmer, Cheryl Jennings, Celsa A Spina, Ann Wiegand, Alan L Landay, Robert W Coombs, Douglas D Richman, John W Mellors, John M Coffin, Ronald J Bosch, David M Margolis (August 13, 2005). "Depletion of latent HIV-1 infection in vivo: a proof-of-concept study". Lancet 366 (9485): 549-55. doi:10.1016/S0140-6736(05)67098-5. http://www.ncbi.nlm.nih.gov/pmc/articles/PMC1894952/. Retrieved 2012-05-09.
- ↑ "Proof of concept, In: Wikipedia". San Francisco, California: Wikimedia Foundation, Inc. December 27, 2012. Retrieved 2013-01-13.
- 1 2 3 "prototype, In: Wiktionary". San Francisco, California: Wikimedia Foundation, Inc. December 8, 2013. Retrieved 2014-01-03.
- ↑ "field-test, In: Wiktionary". San Francisco, California: Wikimedia Foundation, Inc. August 5, 2012. Retrieved 2013-01-13.
- ↑ A. Liu; I. Gorton (March/April 2003). "Accelerating COTS middleware acquisition: the i-Mate process". Software, IEEE 20 (2): 72-9. doi:10.1109/MS.2003.1184171. http://cin.ufpe.br/~redis/intranet/bibliography/middleware/liu-cots03.pdf. Retrieved 2012-02-15.
- ↑ Rhea Wessel (January 25, 2008). "Cargo-Tracking System Combines RFID, Sensors, GSM and Satellite". RFID Journal: 1-2. http://www.rfidjournal.com/article/pdf/3870/1/1/rfidjournal-article3870.PDF. Retrieved 2012-02-15.
- ↑ P. Suresh, C. Rao, M.D. Annable and J.W. Jawitz (August 2000). E. Timothy Oppelt. ed. [http://www.afcee.af.mil/shared/media/document/AFD-071003-081.pdf#page=108 In Situ Flushing for Enhanced NAPL Site Remediation: Metrics for Performance Assessment, In: Abiotic In Situ Technologies for Groundwater Remediation Conference]. Cincinnati, Ohio: U.S. Environmental Protection Agency. pp. 105. http://www.afcee.af.mil/shared/media/document/AFD-071003-081.pdf#page=108. Retrieved 2012-02-15.
External links
This is a research project at http://en.wikiversity.org
![]() |
Development status: this resource is experimental in nature. |
![]() |
Resource type: this resource is an article. |
![]() |
Resource type: this resource contains a lecture or lecture notes. |
![]() |
Educational level: this is a research resource. |
![]() |
Subject classification: this is a Geology resource. |